Conservation of PGR
Motivation
Until now we have seen that there is a strong incentive to conserve plant genetic resources. The key question is, then, which method of conservation is most useful? In the following, the different methods for PGR conservation will be presented and their advantages and disadvantages will be discussed. The conservation of plant genetic resources has both theoretical implications such as the loss of genetic diversity by a given conservation method as well as economic and political consequences such as the cost of conservation and the accessibility by relevant stakeholders (breeders, farmers, lay persons, companies) to the material.
Learning goals
- Understand conservation strategies by being able to distinguish and critically evaluate the advantages, disadvantages, and appropriate applications of ex situ versus in situ conservation strategies.
- Assessing genetic erosion and conservation priorities by understanding genetic erosion, identifying contributing factors, and prioritizing species for conservation accordingly.
- Integration of conservation with breeding practices in discussing the relationship between conservation methods and breeding paradigms, considering biological, ecological, and socioeconomic factors.
- A general bility to practically apply and manage genetic resources, which includes the description of practical methodologies for systematically collecting, evaluating, and managing genetic resources in dynamic gene pools and gene banks.
Practical aspects of PGR conservation strategies
The management of plant genetic resources comprises the integration of all suitable measures to register, describe, collect, evaluate, conserve and provide plant genetic resources.
Conservation Objectives
Before choosing a specific conservation strategy, both general objectives and practical measures need to be clearly defined.
Initially, key questions must be addressed, such as identifying species that require conservation, determining whether local farmers should be involved in conservation activities, and deciding whether national or international experts are best suited to plan and carry out collections. Furthermore, it is important to identify appropriate conservation strategies, select suitable combinations of conservation methods, and assess whether additional detailed studies are necessary before these decisions can be made.
Alongside these general considerations, practical and political aspects are also significant. These include understanding the storage requirements of specific crop species, identifying their mating systems, deciding whether storage is intended for short-, intermediate-, or long-term periods, and evaluating the overall importance of each crop species. Additionally, it is critical to ascertain the geographical localization and accessibility of the genetic resources, determine the most suitable genebanks for their conservation, and identify any necessary or beneficial back-up measures.
Effective management of plant genetic resources (PGR) involves a structured sequence of tasks: registration, description, and evaluation; collection of genetic material; conservation of these resources; provision and documentation of conserved material; and ultimately their use and application. Within these steps, the detailed description and evaluation of crop species and their collections are of special importance. To achieve this, various biological indicators and population genetic parameters are employed, including information about mating systems (e.g., self-fertilizing, outcrossing, clonal or apomictic), genetic diversity within populations, the partitioning of genetic diversity between and within populations, as well as measurements of genetic distance and similarity among populations from different geographic regions.
Overall, decisions about the conservation of plant genetic resources must integrate biological characteristics of the crop species with relevant socio-economic considerations and the political context of the regions where these resources are collected and conserved. Because of their biology, some species are easier to collect, conserve and propagate, than others, and therefore biological variation results in different costs of conservation.
Comparison of ex situ and in situ conservation
A key decision in the conservation of plant genetic resources is whether conservation should be in situ, ex situ, or both. In situ conservation means that the genetic variation is maintained in the location and environment of original occurrence and use, whereas in ex situ conservation, the genetic material is stored elsewhere outside of the original environment. Both approaches have advantages and disadvantages.
They will be summarized in the following overview of different types and methods of ex situ and in situ conservation.
Methods for in situ conservation
Genetic reservoirs
Genetic reservoirs represent geographic areas distinguished by their rich diversity of landraces or crop wild relatives, which are granted a special status of protection due to their highly valuable contribution to biodiversity (Maxted et al., 2008). These reservoirs offer significant advantages by facilitating the dynamic conservation of genetic resources, allowing them to adapt to changing environmental conditions, diseases, and pests. Their geographical coherence or restriction enhances their utility for evolutionary and genetic studies, as plants can persist in their native habitats or cultivation systems. This aspect is particularly beneficial for ‘recalcitrant’ species, which are difficult to conserve through conventional methods. Furthermore, genetic reservoirs enable the straightforward conservation of related wild species within their native environments and offer the opportunity to preserve multiple crop species simultaneously.
However, there are disadvantages associated with genetic reservoirs. The management of these areas often suffers from a lack of experience, and the amount of genetic diversity that can be conserved within each reservoir is inherently limited by the native diversity present. Additionally, the genetic material conserved in these reservoirs is not readily available for immediate use. They are also vulnerable to both natural and human-induced disasters, such as fires, vandalism, urbanization, pollution, wars, and more, which can severely impact their effectiveness and sustainability. Consequently, genetic reservoirs demand a high level of supervision and thorough documentation to ensure their long-term viability and success in conserving genetic diversity.
An outline of genetic reserves in Europe can be found in Rubio Teso et al. (2020).
On farm conservation
On-farm conservation presents a nuanced approach to safeguarding agricultural biodiversity by leveraging both its dynamic capabilities and traditional practices. This method offers significant advantages, particularly in its dynamic conservation model, which actively responds to changing environmental conditions, diseases, and pests. It is inherently adaptable, reflecting changes in agricultural practices and enabling the preservation of traditional landraces of crop species. Furthermore, on-farm conservation facilitates the straightforward conservation of related wild species and breeding stocks, ensuring a diverse genetic pool for future agricultural resilience and development.
However, this conservation strategy is not without its challenges. Its susceptibility to changes in agricultural practice can be a double-edged sword, requiring the maintenance of traditional agricultural systems that may necessitate financial incentives or subsidies to sustain. The approach is limited by a general lack of management experience (or traditional knowledge), making its implementation and ongoing operation more complex and potentially less efficient. Another significant limitation is the reduced level of genetic diversity that can be preserved on any single farm. To achieve a sustainable outcome of on-farm conservation efforts, collaboration across several farms in diverse regions is essential, which complicates logistics and increases the necessity for efficient coordination. Additionally, there is a risk of confusion with participatory plant breeding strategies, in which farmers select genotypes based on their own personal preferences, which could interfere with the overal goals of conservation and dilute the focus and effectiveness of on-farm conservation efforts.
In summary, on-farm conservation represents a balanced strategy for preserving agricultural biodiversity, because it attemtps to integrate the complexities of modern environmental challenges with traditional agricultural values. However, it demands thoughtful implementation, robust support systems, and clear separation from other agricultural practices to fully realize its potential benefits and mitigate its disadvantages.
Seed savers associations such as Genbänkle e.V. ((Website)[https://www.genbaenkle.de/]) or Pro Specie Rara e.V. (Website) attempt to maintain on-farm conservation by accounting for the above considerations. A survey on on-farm conservation in Europe is provided by Raggi et al. (2024).
Home gardens, fruit orchards
Home gardens and fruit orchards represent a different approach to biodiversity conservation (Korpelainen, 2023). They blend the preservation of genetic diversity with the cultivation of a wide range of plant species, including rare crops, fruits, vegetables, medicinal plants, spices, and fruit trees. This method offers the advantage of dynamic conservation, because the cultivated plants can respond to changing environmental conditions, diseases, and pests and therefore co-evolve with their environment, similar to on-farm conservation. It facilitates the conservation of traditional landraces (or heirloom varieties), which are crucial for maintaining genetic diversity and cultural heritage.
However, home gardens also have some challenges. The are susceptible to changes in horticultural practices, which can impact the effectiveness of biodiversity conservation efforts. Additionally, there is generally little broad experience available for the management of such diverse and ecologically complex systems, and it is mainly based on the personal experiences and practices of each gardener. This lack of expertise necessitates the preservation of traditional agricultural practices, which may require financial support in the form of subsidies to encourage and maintain these practices.
In summary, while home gardens and fruit orchards offer a promising avenue for the conservation of a wide array of plant species and the maintenance of genetic diversity, they also present challenges that need to be addressed. These include the need for adaptation to changing horticultural practices and the requirement for specific knowledge and financial support to sustain traditional agricultural practices.
Ex situ conservation
Seed storage
Seed storage is a widely applied method within ex situ conservation of crop plants and their wild relatives due to several practical advantages (Smith, 2003). It is efficient, reproducible, and suitable for short-, intermediate-, and long-term preservation of genetic material. This approach allows the conservation of extensive genetic diversity within a single species, facilitating straightforward access to the material for characterization, evaluation, and subsequent use. Additionally, seed storage generally demands a relatively low maintenance effort, making it cost-effective and manageable for conservation purposes. Most major ex situ genebanks are predominately seed storages.
However, seed storage also presents several challenges. Certain seeds, known as recalcitrant seeds, cannot withstand conventional storage conditions and thus pose significant conservation difficulties. Moreover, the evolutionary processes of the stored genetic material, especially concerning pathogen and herbivore resistance, effectively come to a halt during storage, limiting the adaptive potential of the conserved genetic resources.1 Genetic diversity may also be progressively lost due to the regeneration procedures needed to maintain seed viability. Lastly, by concentrating on individual target species, seed storage methods often overlook associated plant species from the original collection sites, which might also harbor valuable genetic diversity.
1 For a discussion of this issue in a more general context, see Mattana et al. (2025).
In vitro storage
In vitro storage is a valuable method in ex situ conservation, particularly beneficial for long-term conservation of recalcitrant, sterile, and clonal plant species. It offers straightforward access to plant material for subsequent use and evaluation, thereby facilitating efficient characterization and application.
However, this method carries the risk of somaclonal variation, potentially altering the genetic identity of conserved material. Furthermore, individual species typically require specific tissue culture protocols, making the conservation process labor-intensive and complex. Lastly, maintaining in vitro cultures demands relatively extensive technological resources and incurs high maintenance costs. These costs can be reduced by developing protocols by cryopreservation that can provide very long storage in comparison to classical in vitro culture on agar plates and can be made cost-efficient with modern storage containers (Nagel et al., 2024).
DNA storage
DNA storage provides a comparatively simple and inexpensive method for conserving plant genetic resources, making it particularly attractive when resources are limited.
Despite this, it currently presents significant practical limitations. Most notably, regeneration of whole plants from stored DNA is not yet feasible, severely restricting the practical usability of conserved genetic material. Additionally, complications associated with gene isolation, cloning, and gene transfer techniques limit the immediate applicability of stored DNA, making DNA storage primarily a complementary rather than standalone method for conservation.
Pollen storage
Pollen storage offers another relatively straightforward and cost-effective approach for conserving genetic resources. It allows storage of genetic material efficiently and inexpensively, often enabling the conservation of mixtures derived from multiple individuals, thus preserving a broader genetic base.
Nevertheless, pollen storage has notable drawbacks. Regeneration procedures for pollen typically require specific protocols tailored individually for haploid plant regeneration and subsequent diploidisation, adding complexity to the conservation process. Additionally, since pollen contains only paternal genetic material, maternal genetic diversity is not conserved, limiting the representation of the full genetic diversity of the species.
Field gene banks
Field gene banks provide a suitable conservation method for species that cannot be conserved using conventional seed storage techniques, such as recalcitrant species. They offer direct access to conserved material for characterization, evaluation, and utilization, and allow continuous evaluation of plants during the conservation period. Many perennial cultures such as grapevine, fruit trees and other fruits are conserved in field gene banks.2
2 E.g., the German fruit tree genebank in Dresden-Pillnitz (Website)
However, field gene banks expose plant material to environmental hazards such as insect herbivores, diseases, and vandalism, thus posing significant risks to conservation success. Additionally, they require substantial land areas even for relatively small population sizes and incur high ongoing maintenance costs, making them resource-intensive conservation options.
Botanical gardens
Botanical gardens play a distinct role in the conservation of genetic resources, particularly focusing on wild plant species without significant commercial value. They function effectively as educational and public demonstration gardens, offering a practical context for public awareness and teaching about biodiversity.
Nevertheless, botanical gardens typically require considerable space, significantly limiting the number of species and genetic diversity that can be conserved effectively—often maintaining only single or few individuals per species. Furthermore, botanical gardens are associated with high maintenance costs due to the extensive labor and resource input needed to sustain diverse plant collections.
Which conservation strategy?
With the different options for PGR conservation known, a strategy needs to be defined. A possible strategy for the utilization of genetic diversity in breeding programs and the use conserved genetic resources in in situ and ex situ collections is shown in Figure 1.
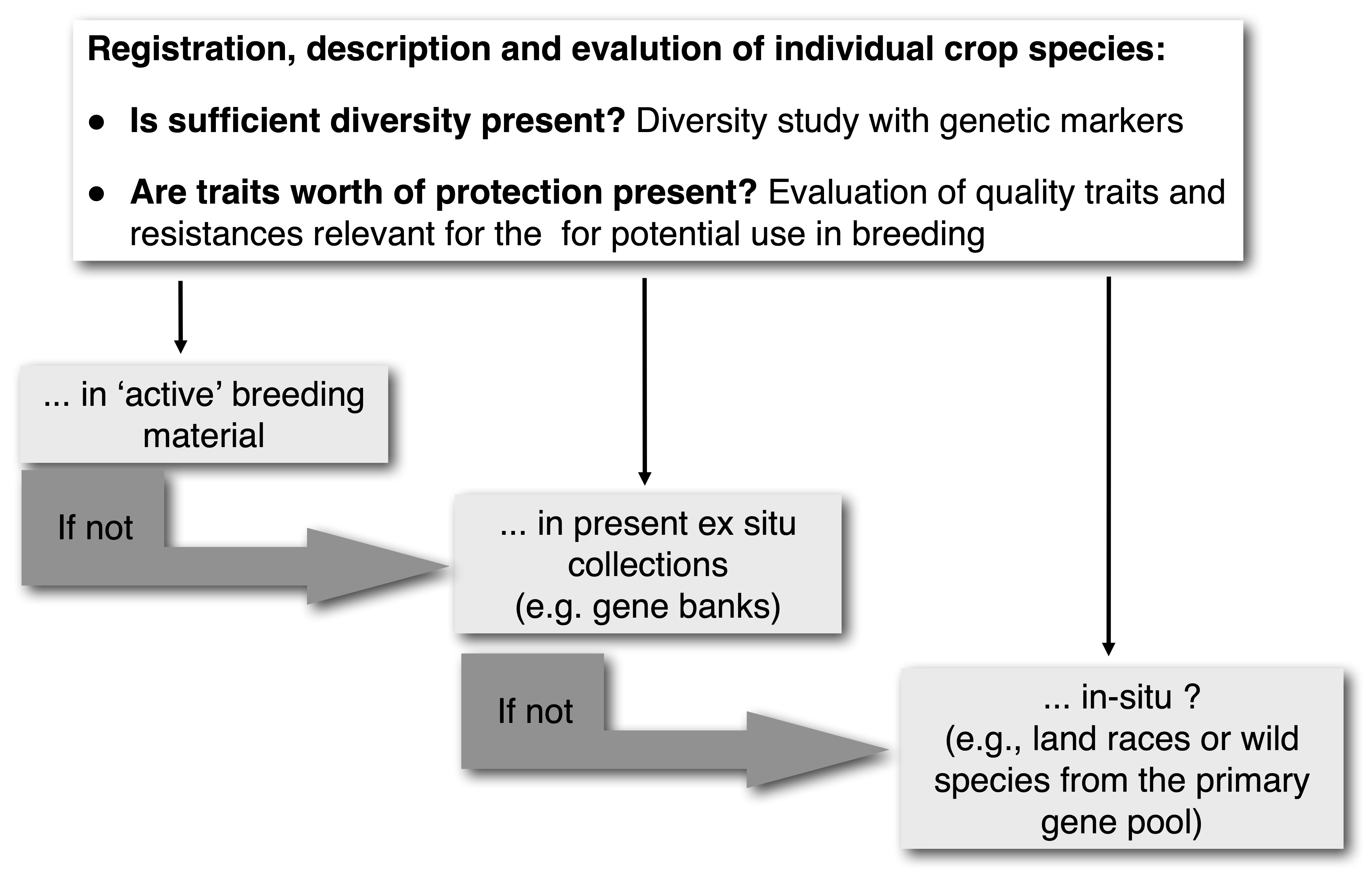
The development of the PGR conservation movement was accompanied by highly controversial discussions on the best strategy to conserve PGR. In particular, the discussions centered around the question of whether ex situ (in genebanks) vs. in situ (on the field) conservation is more appropriate and sustainable. At first glance, the discussion is purely scientific in nature, but in fact affects how agriculture and plant breeding will be done in the future.
Briefly put, there is a match between breeding strategies and conservation strategies as shown in Table 1.
Feature | Breeding-oriented | Conservation-oriented |
---|---|---|
Trait | Simple traits (Resistance genes) | Complex, adaptive traits |
Breeding method | Introgression of alleles | Composite crosses of diverse genotypes |
Selection by | Plant breeders (Participative breeding) | Nature and farmers |
Conservation | ex situ | in situ |
The conflicts followed professional lines:
- Agriculturalists and geneticists
- This group favored resistance breeding which depends on the ex situ conservation of genetic resources. A very rigid scientific framework was available (quantitative genetics and selection theory) and successes are easy to demonstrate.
- Conservationists and environmentalists
- They favored participative breeding approaches and in situ conservation. However, no good theoretical concept was (and is) available to investigate questions like “What diversity is important?” or “What is the dynamics of diversity over space and time?”
Differences in breeding paradigms
The breeding paradigms can be differentiated between the single resistance gene (resistance genes) versus the polygenic trait (adaptive traits) concepts. The former concept originated from the fact that the first type of resistance found in plants was monogenic. This led to the famous lock-and-key relationship model of pathogens and their host plants as proposed by Flor (1956). On other words, resistance against pathogens for a long time was considered to be race-specific. Since this concept was so successful and resistance breeding so important, there was no serious search for alternative concepts to single gene resistance.
Pyramiding, i.e., the combination of different resistance genes in a single variety selects for combinations of single gene resistances that provided resistance at the time of breeding.
The single-gene concept also had a practical aspect because breeding for polygenic resistance is impractical in monocultural agricultural systems. With polygenic resistance, pathogens are not killed entirely, and a limited yield loss is tolerated by the farmer. For this reason, one way to differentiate the two concepts is:
- Single gene resistance \(\Rightarrow\) Block any infection
- Polygenic resistance \(\Rightarrow\) Reduce the rate of infection
On the other hand, single gene resistance is expected to be less dureable from an evolutionary point of view (i.e., pathogens can overcome the resistance easily), whereas polygenic resistance requires adaptation to a view genes simultaneously, which is difficult to achieve.
Socioeconomic aspects of breeding paradigms
Another aspect results from the fact that farmers in developed countries can afford the expensive seeds produced by breeding companies, but can not afford seed and yield losses. Since most developed countries are located in the Northern hemisphere, pathogens are less active due to a temperate climate, and resistances can persist longer. Furthermore, there is a greater emphasis on food quality. Finally, there are uniform, high-yielding farming systems in the North that support the scientific breeding, ex situ conservation paradigm.
In contrast, peasant and other small-scale farmers in developing countries accept some yield loss, but reward high food security provided by polygenic resistance.
Arguments in favor of in situ conservation
Although the agronomist Jack Harlan was not a strong supporter of in situ conservation, but he suggested to integrate in situ conservation approaches. Harlan called this adaptation syndromes resulting from automatic selection via adaptation through natural selection. Accordingly, the high diversity of landraces is explained to result from local adaptation. To quote Harlan (1975),
Landraces have a certain ‘genetic integrity’. They are recognizable morphologically; farmers have names for them and different landraces are understood to differ in adaptation to soil type, time of seeding, date or maturity, height, nutritive value, use and other properties. Most important, they are genetically diverse. [They are] balanced populations - variable, in equilibrium with both environment and pathogens, and genetically dynamic …
Another important line of arguments was that modern pure line breeding leads to an imbalance between the crop and its environment, which requires a high-input agriculture. Since many seed companies sell packages of seeds and pesticides, such an imbalance may be an increased side effect to increase profit.
Modern plant breeding also is supposed to lead to boom and bust cycles because resistance breakdown occurs frequently, and new varieties constantly need to be generated. Therefore, such an approach leads to a ‘treadmill’ of new varieties. One important counterargument against this view was the observation that pathogen epidemics occurred throughout agricultural history (e.g., ergotism). Another point of criticism of in situ conservation was the fact that they were not incorporated into modern breeding programs and therefore received little support from the plant breeding companies.
Arguments in favor of ex situ conservation
The key arguments of the supporters of ex situ conservation combined with scientific plant breeding group was that the green revolution varieties produce a higher yield and help to avoid population pressure and to prevent starvation on a global scale.
It was an important observation that quite frequently introduced elite varieties were more superior than locally adapted landraces (with the exception of extreme conditions, under which local varieties give more syield).
Furthermore, genecological experiments (i.e., letting plants to adapt to local environmental conditions such as in participative breeding) never gave higher yields than modern elite varieties and did not result in better varieties for wide use. This is an important point because land races were considered as reserves of genetic diversity but also as a material stock for coevolution with pathogens.
Based on a central research premise of ecology that diversity promotes stability, a genecological approach often was supported because of a misunderstanding of evolutionary theory by proponents of “alternative” breeding approaches.
Differentiation versus adaptation:
Landraces differ in many characteristics from each other. Which of these result actually from adaptation or instead represent just ‘neutral’ divergence:
- ears with and without awns
- kernel color vs. kernel size
Genetic drift (the random fixation of alleles) instead of natural selection may have caused such differences as well. Therefore, they are not necessarily adaptive.
Adaptability is limited by the effective population size:
Since pathogens usually have much larger population sizes than their plant hosts, they can evolve faster and outcompete any coadaptation of plants to their pathogens. For this reason, the introduction of a new and resistant variety may be more successful than letting the local genotypes coevolve with their pathogens.
- Limited local diversity:
- Even though the genetic diversity of a crop species may be high in total, the diversity available for a particular farmer may be limited.
- Wrong selection:
- Strong selection for easily scorable traits such as morphological traits that may or may not be important for yield strongly reduce genetic variation in land races and future potential for genetic improvement.
Another argument against landraces stated that market forces demand high yield. Since pesticides and fertilizer were relatively cheap at the beginning of the green revolution, breeders did not select for low-input varieties, and made very good profit by selling plants, pesticides and fertilizer together as a package.
Furthermore, the preferred single-gene resistances are easier to understand on a mechanistic level and more manageable (and therefore cheaper) than complex traits.
The relationship between breeding and conservation
Single gene resistances are easily accessible and surveyble in ex situ stocks. In contrast, polygenic traits are difficult to monitor. An ex situ conservation approach is more practical for breeders; interestingly, surveys showed that breeeders in fact favor their competitor’s elite material over gene bank material because the latter does not coevolve with pathogens and has many undesirable alleles, that has been removed in elite material.
These considerations suggest that ecologist lost the debate of ex situ vs. in situ conservation both scientifically (little research going on) and economically. Nevertheless, the in situ conservation approach is still strongly promoted by grassroot organisations, NGOs and national developmental agencies under the terms participatory breeding, evolutionary gardens and composite crosses that all support coevolutionary relationships.
One example is the Open Source Seeds movement.3. However, such grassroots movements so far have little economic impact.
Overview of ex situ conservation
The current state of ex situ gene banks
The state of ex situ gene banks is summarized in the 3rd FAO Report on the State of World’s Genetic Resources for Food and Agriculture (The Third Report on The State of the World’s Plant Genetic Resources for Food and Agriculture, 2025)4:
4 The following numbers are from the second report, FAO (2010)
- 1,750 individual gene banks worldwide
- 130 hold more than 10,000 acessions each
- There are substantial collections in the 2,500 botanical gardens
- Genebanks are on all continents, but there are relatively fewer in Africa
- The CGIAR collections are among the largest ones
- Currently there are about 7.4 million accessions
- Only 1.9 - 2.2 million accessions are distinct (about 25-30% of the total)
- Of the most important crops, 4.6 million accessions are stored
- National gene banks harbor 6.6 million accessions
- 45% of national collections are only in seven countries, increased concentration into fewer countries
The geographic distribution of gene banks is shown in Figure 2.
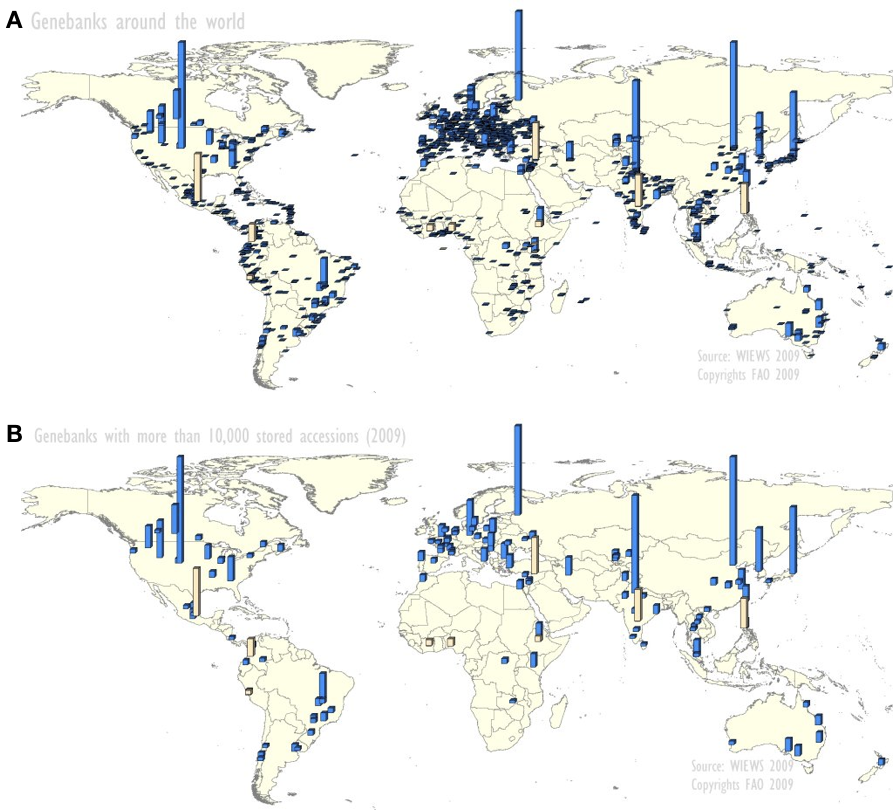
The collection activities leading to an increase of genebank accessions is shown in Figure 3.
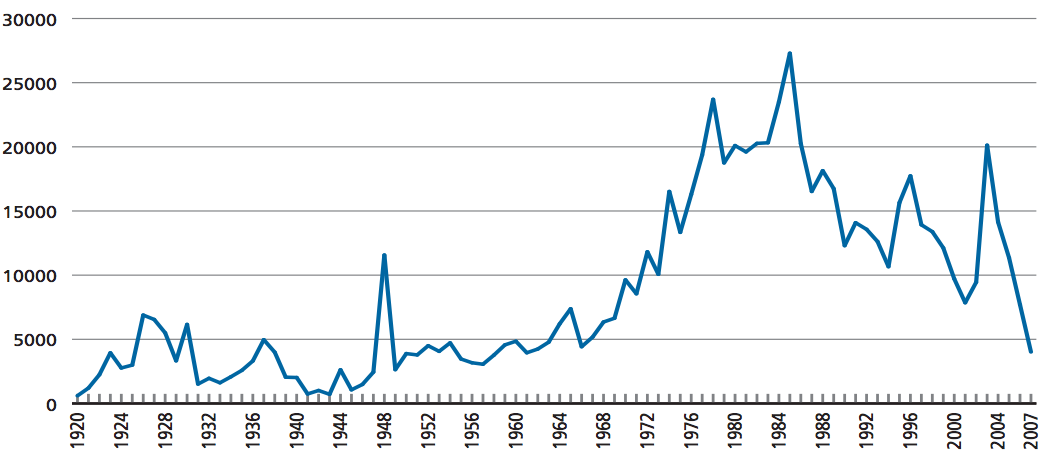
The different types of accessions and types of crops collected are shown in Figure 4.
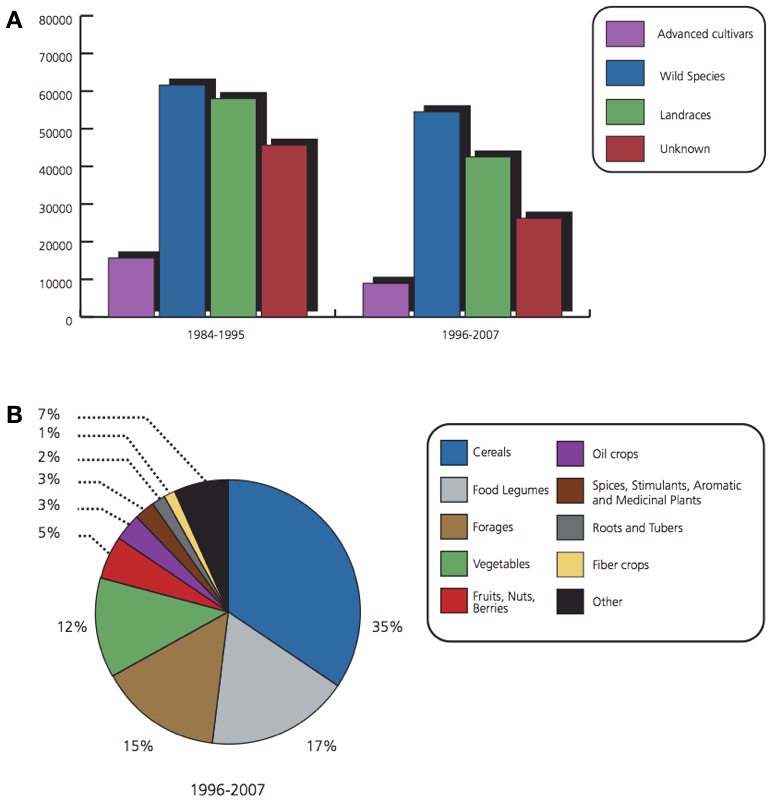
The sizes of global gene bank collections for the major crops are shown in Figure Figure 5.
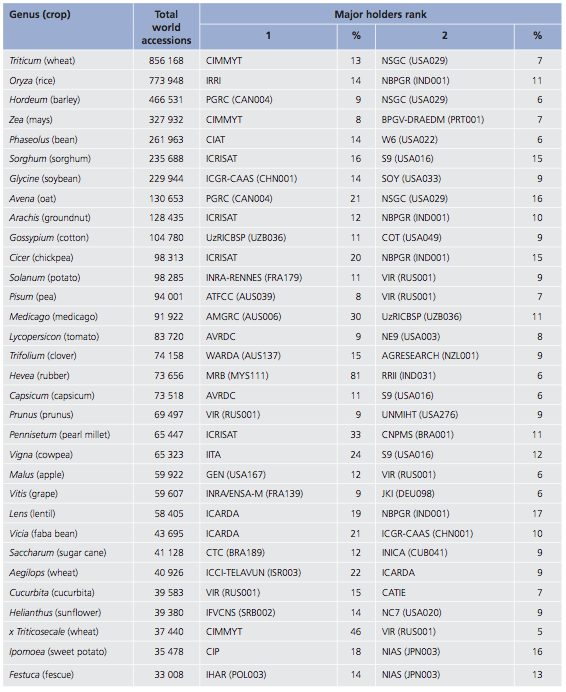
Types of ex situ gene banks
The main types of ex situ are differentiated whether whether they conserve living plant materials, plant tissues, or seeds. The type strongly depends on the biology of the plant species to be conserved (Figure 6}.
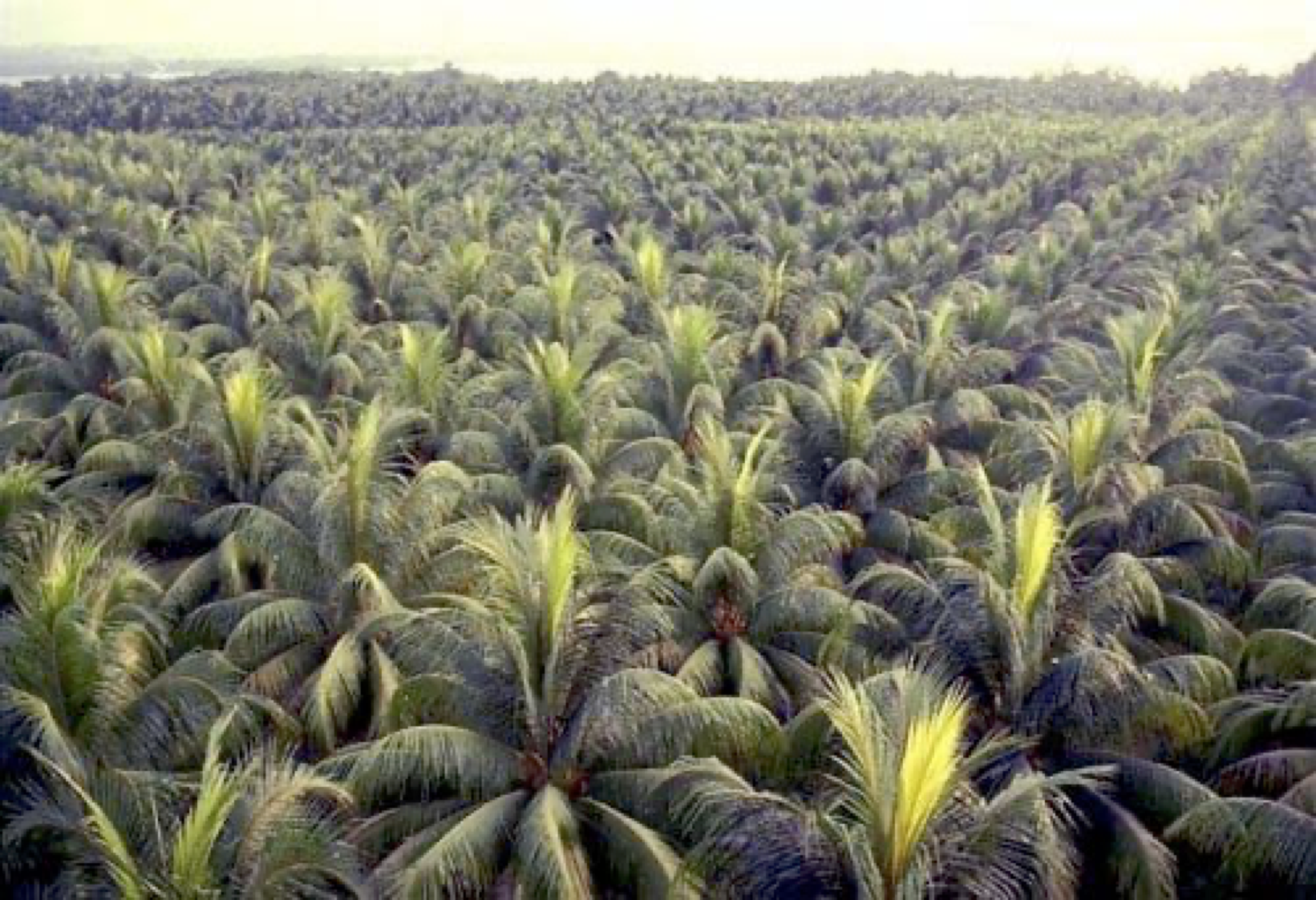
Advantages and disadvantages of ex situ gene banks
The advantage of gene banks is that genetic resources are collected at a few locations, where they may be protected well and efficiently analyzed and researched. However, the concentration at one location may endanger the collection because of potential disasters.
Open air genetic repositories or genebanks are endangered by environmental damage. Figure 7 shows a strongly damaged genebank of maracuja in Taray, Peru after a big flooding.
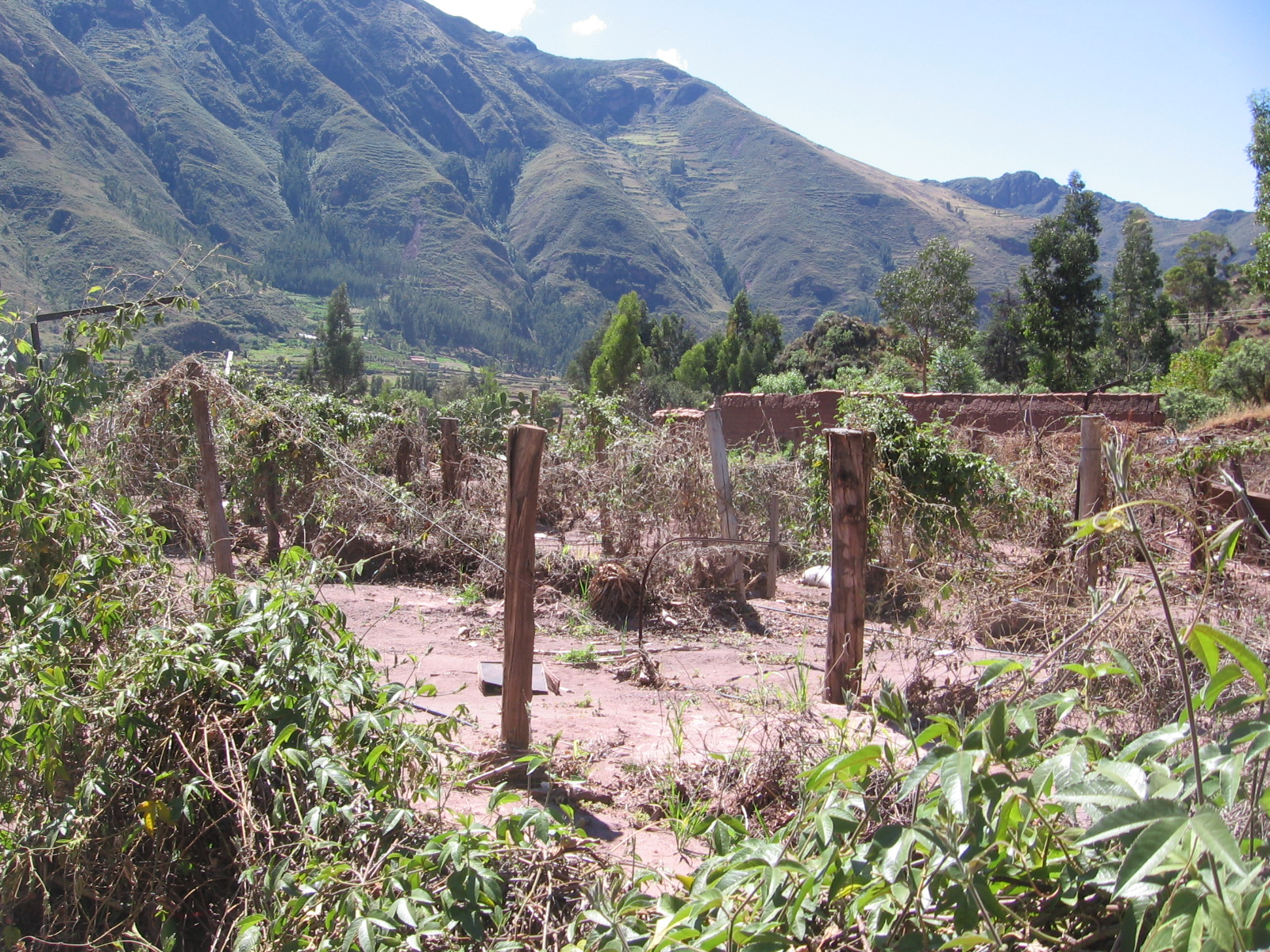
Figure 8 shows a genebank of quinoa in Camacani, Peru. The building is a simple hut with an imperfect electrical wiring. There is also no air conditioning. The temperatures rise and fall every day by a big amplitude which has a strong effect on germination ability.
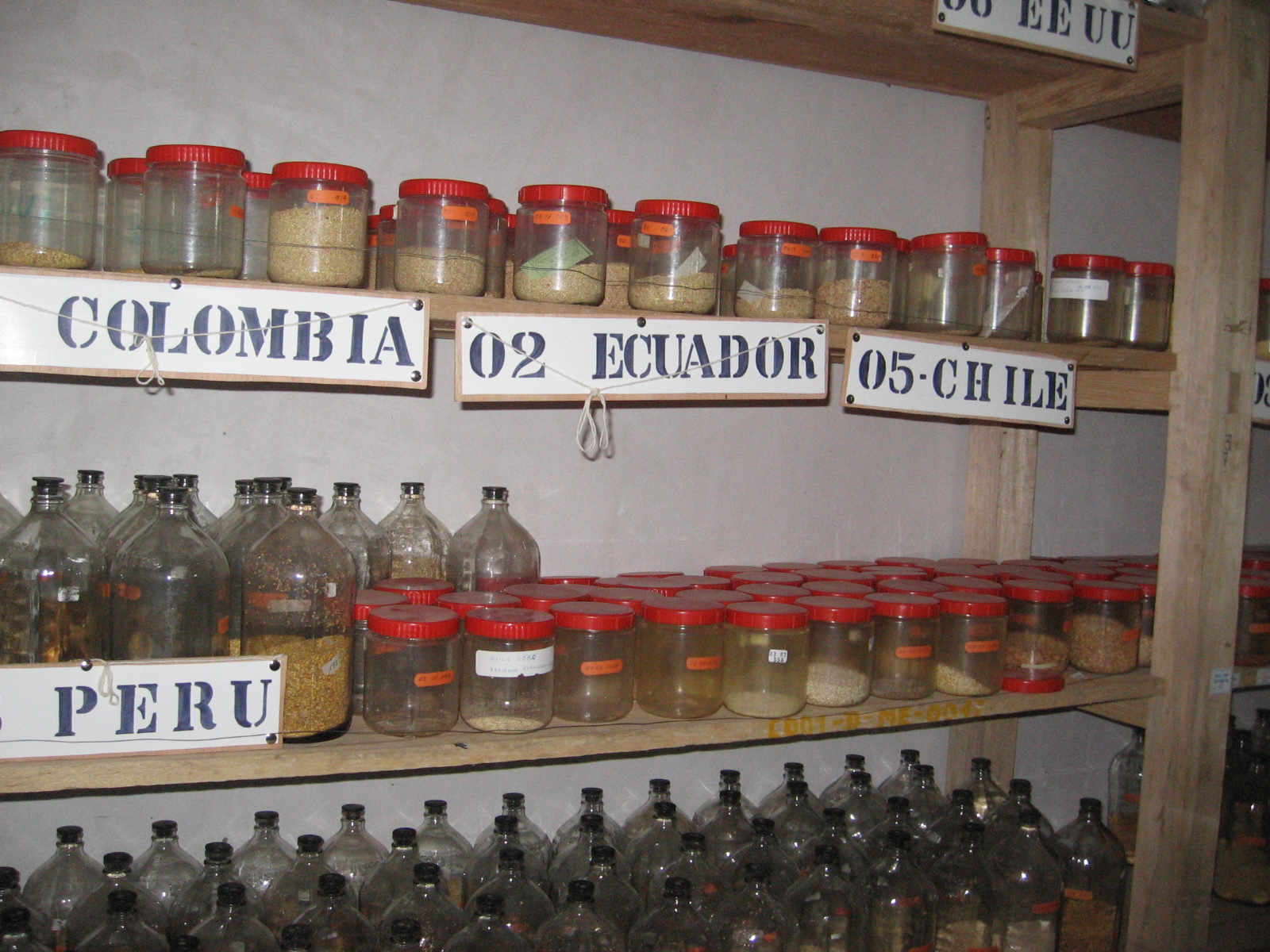
Global Seed Vault in Svalbard
The most recent addition to the global system of gene and one of the more spectacular gene banks is the ‘ice bunker’ in Spitsbergen. The so-called Global Seed Vault in Svalbard has the mission to preserve seeds in an underground facility to provide insurance against loss of seeds in case of major catastrophies.5 It was established in 2008 and is funded by the Norwegian government and other donors. The content of the Seed Vault are duplicates from other gene banks. There is no regeneration or duplication of the material at the site.
5 http//www.nordgen.org/sgsv
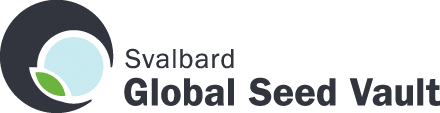
The GSV is located in a former iron mine that has been renovated and adapted to storage of a seed vault.
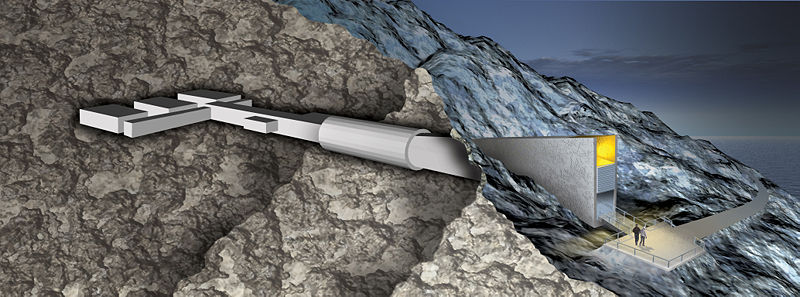
Collection and evaluation of PGR in ex situ conservation
Both the collection as well as the subsequent evaluation of PGR in ex situ conservation requires careful planning. The collection sites have to be chosen carefully to represent the diversity of environments and the geographic rance in which a crop occurs in order to capture as much genetic diversity as possible. The subsequent evaluation needs to consider in which environments a collection should be grown and which traits should be evaluated.
Wild and cultivated barley in Syria and Jordania
As an example, the systematic collection and evaluation of a wild and cultivated barley collection is shown in the following. Both wild and cultivated barley are ecologically highly variable species that likely have locally adapted to the different environments. For the establishement of a systematic collection, both wild and cultivated barley were collected a numerous, environmentally diverse sites in Syria (Figure 11).
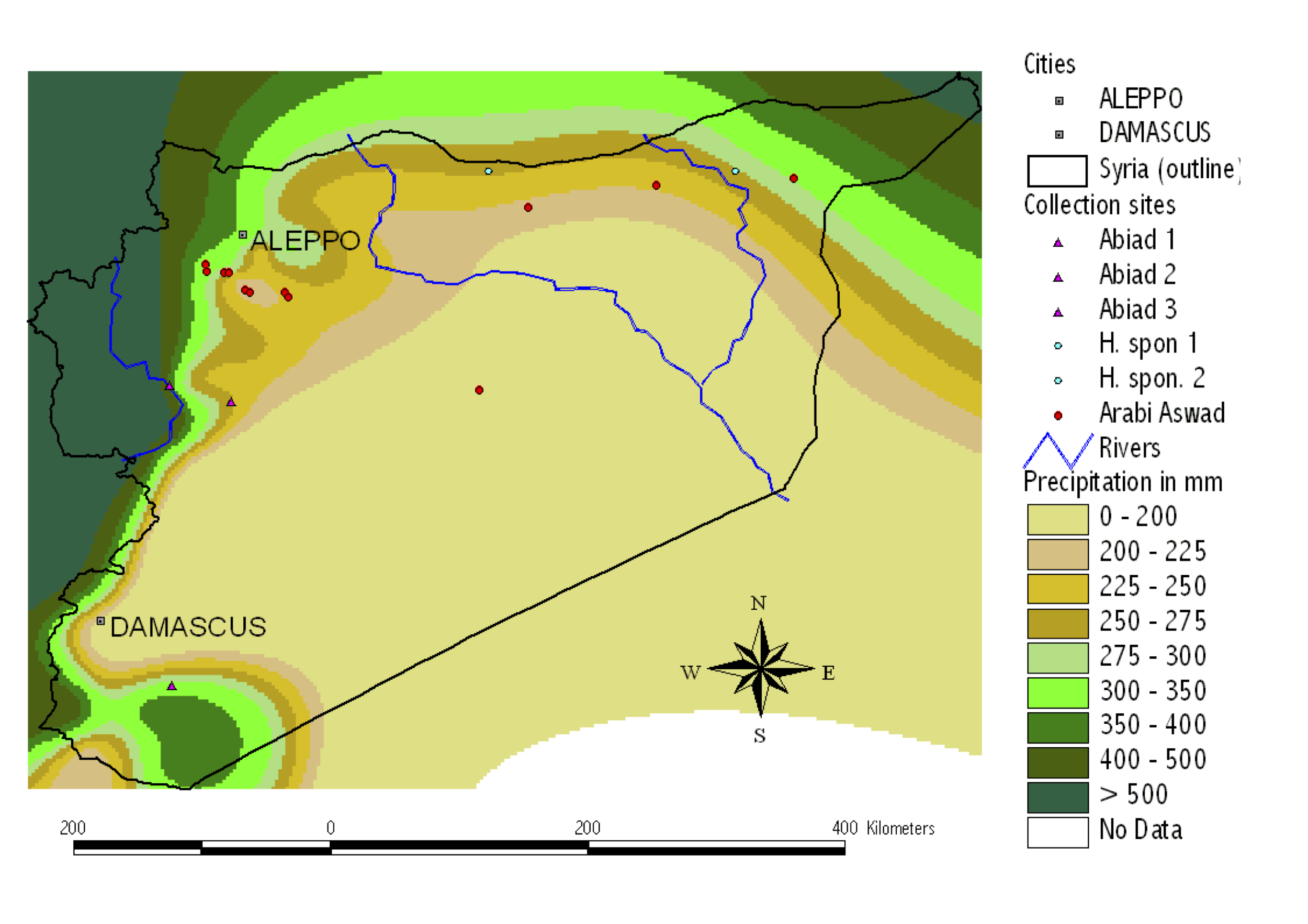
The barley accessions collected in Syria were genotyped with 25 simple sequence repeat (SSR) genetic marker loci, and diversity statistics were calculated (Table 2) to estimate the diversity present in the collection. The shows differences in the extent and type of genetic diversity between the various locations.
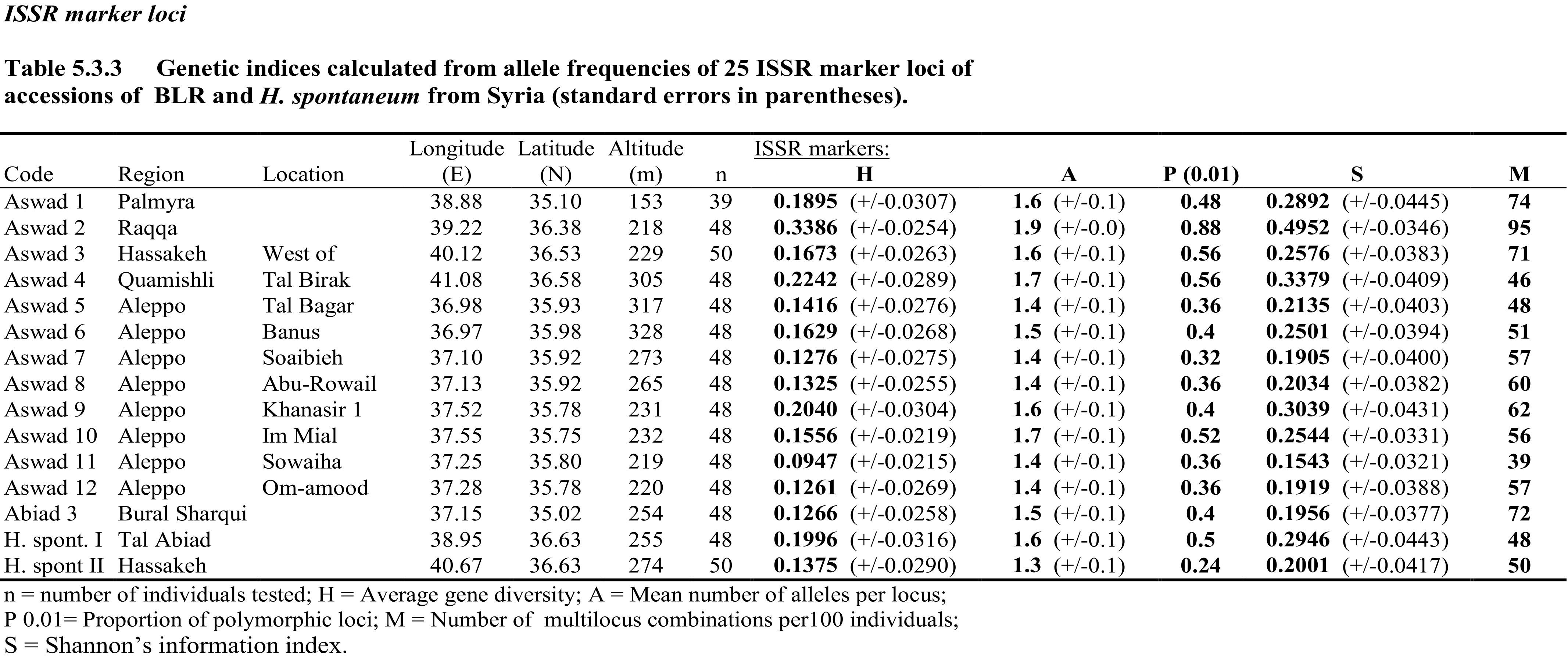
In addition to the genetic analysis, it is also possible to identify useful accessions from collection by describing their phenotypic characteristics. Unfortunately such characteristics are rarely obtained in a systematic fashion for gene bank accessions, therefore experiments have frequently to be carried out again for particular purposes. One example is a study of barley land races (Table 3)
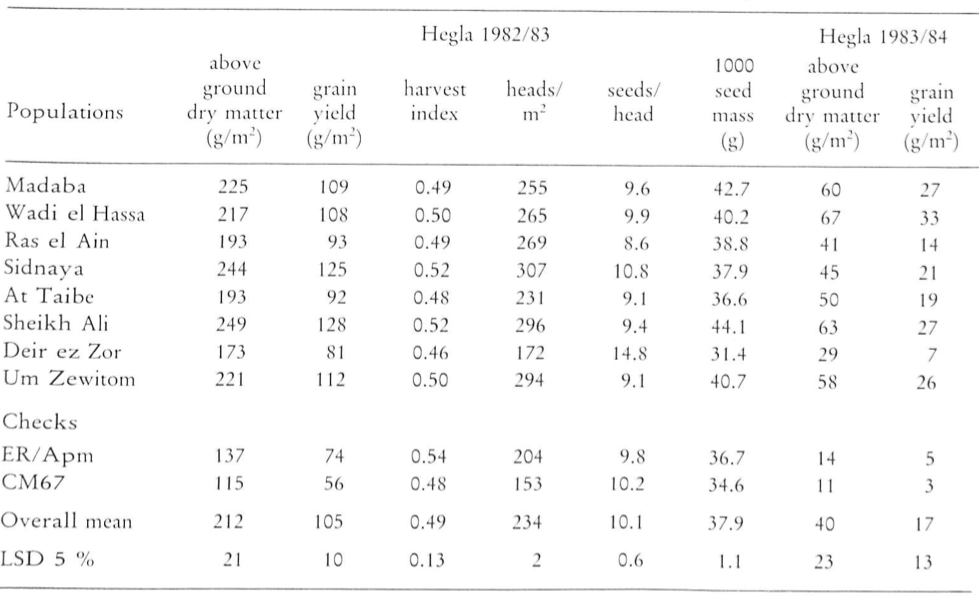
Figure Figure 12 shows the geographic origin of the accessions from Jordania.
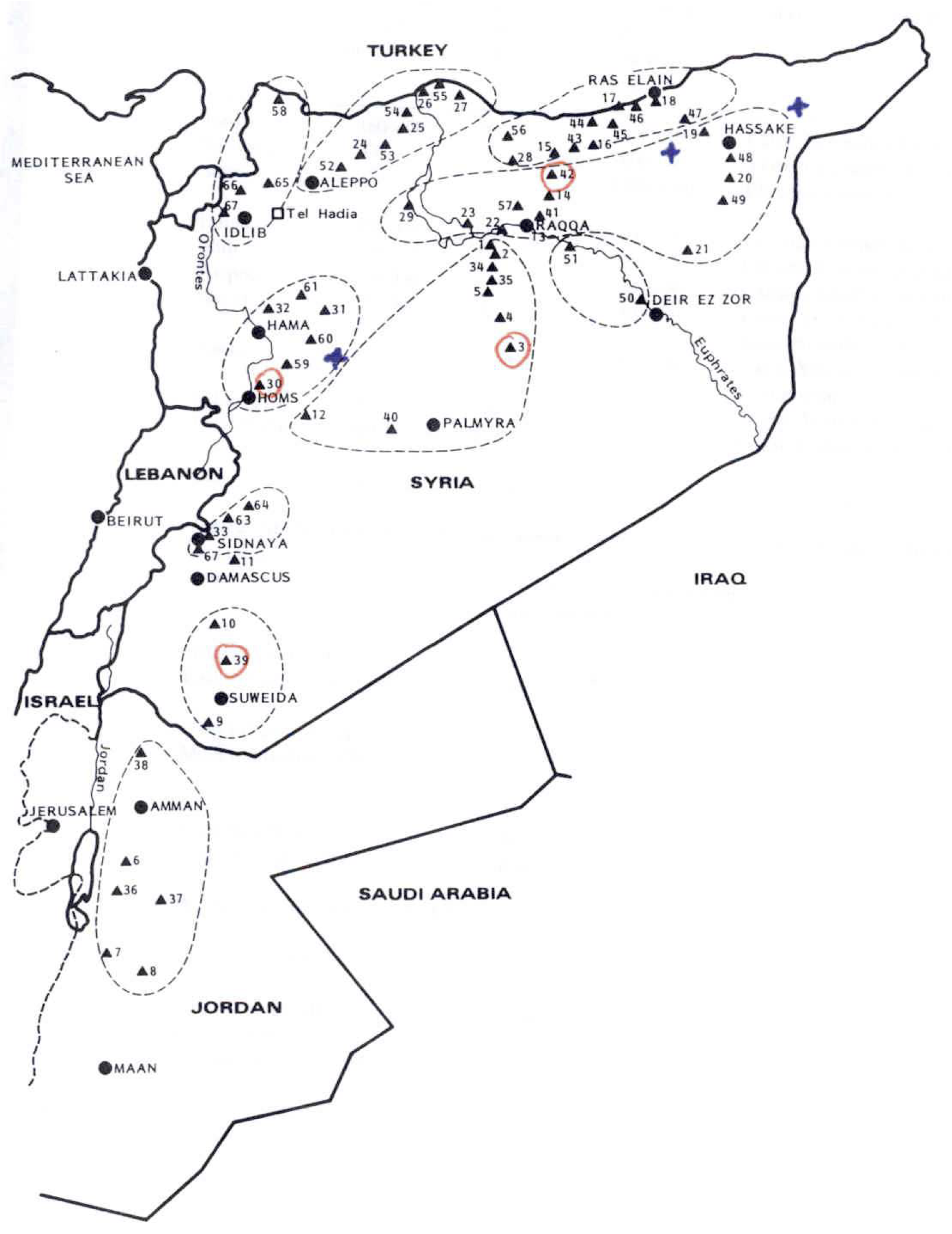
Consequences of the description analysis of PGR
The detailed description and analysis of plant genetic resources (PGR) play a critical role in shaping conservation strategies.
By assessing genetic diversity within and between crop populations, priorities for conservation can be redefined to focus on species or varieties that are most at risk or hold the greatest potential for future use. This process also enables the evaluation of the risk of genetic erosion, helping to identify vulnerable resources that require urgent conservation measures. Furthermore, it can reveal gaps in existing collections, leading to efforts to make them more comprehensive and representative of the overall diversity. In some cases, such analyses may justify the initiation of entirely new collection efforts in underrepresented regions or for neglected species.
Ultimately, the insights gained from PGR description may prompt a reassessment and refinement of the overall conservation strategy to ensure it aligns with both scientific evidence and practical needs.
The risk of genetic erosion
For crop species maintained in situ, the risk of genetic erosion, i.e., the loss of genetic variation, can be estimated with the developed by ??
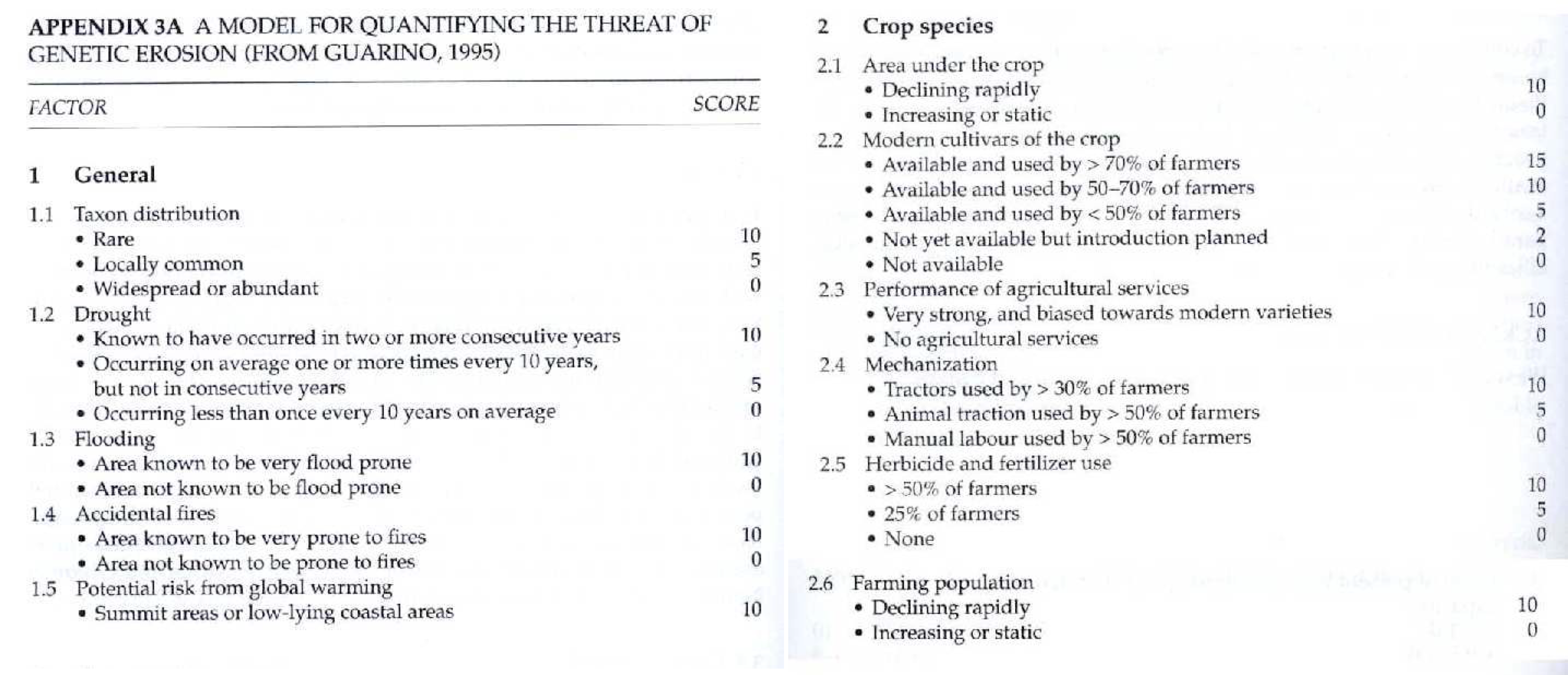
Which species have a high risk of erosion and require that they are conserved with high priority? Examples of such groups of species are:
- Tropical and subtropical fruit species in South East Asia, Central and South America
- Unique crops of the high Andes such as Quinoa, Amaranthus, Oxalis, Ullucus and Tropaeolum-Species.
- Traditional medicinal plants from South and South East Asia as well as from other tropical countries
- Leaf vegetables from small farms in all regions of the world.
The loss of genetic variation in gene banks has been analyzed, for example in barley. In this study, genetic diversity was measured as average gene diversity, \(H\). Samples of barley land races from the North of Syria from a current collection in Syria from 1997 and 1998, as well as from gene banks that were stored for 10, 39 and 72 years (Figure 14; Parzies et al. (2000)).
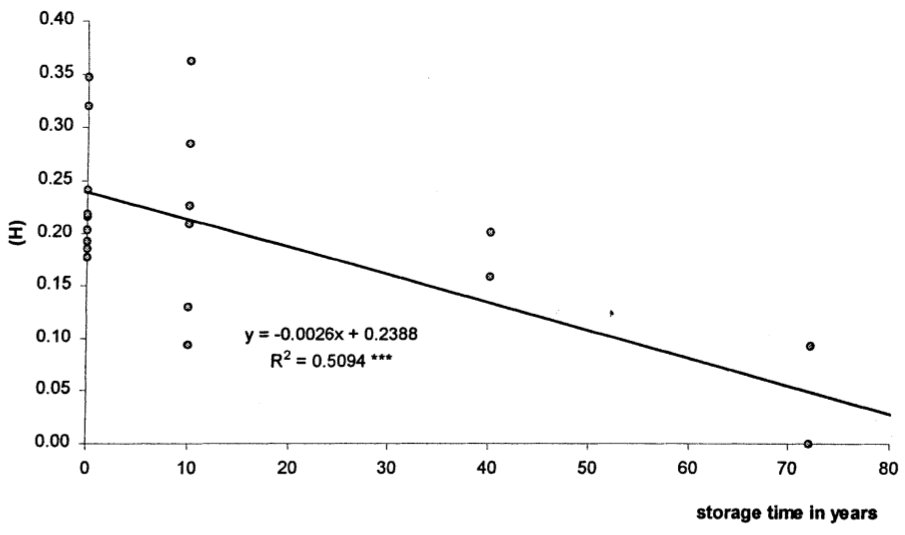
The cause of genetic erosion is a loss of genetic variation by genetic drift due to a small effective population size, \(N_e\). It can be estimated from the observed decay of diversity, \(H\). The remaining diversity, \(H_t\) after \(t\) generations is calculated as \[\begin{equation} \label{decay1} H_t = H_0(1-\frac{1}{2}N_e)^t \end{equation}\] which can be reformulated to \[\begin{equation} \label{decay2} log(H_t) = log(H_0) + t \times log(1-\frac{1}{2}N_e). \end{equation}\] The regression of \(log(H_t\) on \(t\) can be calculated and the slope of the regression allows to estimate \[\begin{equation} \label{decay3} log(1-\frac{1}{2}N_e), \end{equation}\] from which \(N_e\) can be derived.
The observed and expected (under an infinite population size) decay of the heterozygosity for the barley data is shown in Figure 15. The calculations were made with the assumption of a rejuvenation cycle each 5.3 years, no loss in germination ability and the inclusion of all markers.
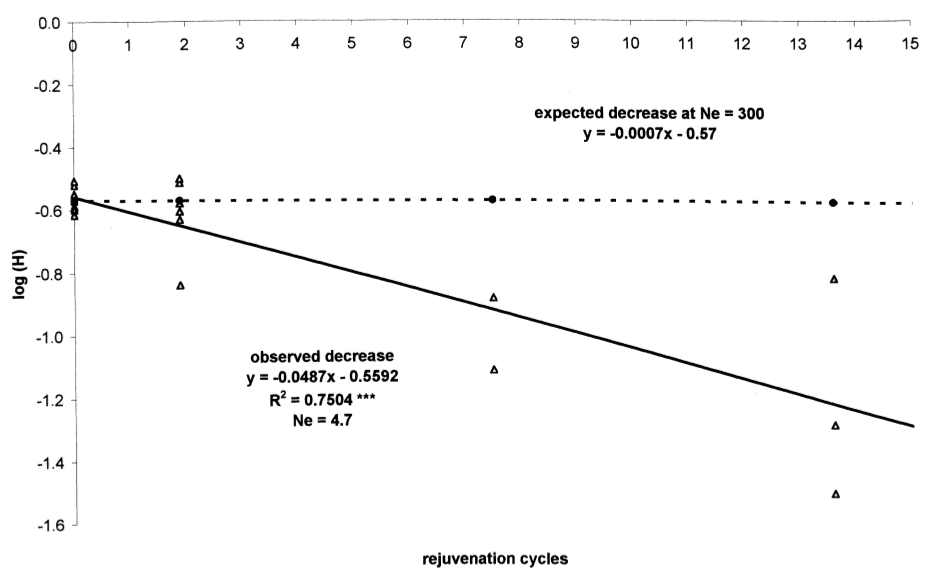
To summarize, the diversity of gene bank collections of barley decays over many years because populations pass through genetic bottlenecks. In outcrossing species, the decay of diversity is even more significant than in self-fertilizing species. One option to counter this effect is to increase plot size used for rejuvenation or to rejuvenate the seeds less frequently. Alternatively, \(N_e\) can be increased to \(N_e=2N-1\) by targeted crosses.
Overview of in situ conservation
In situ conservation as defined according to the German Ministry of Agriculture (2000)
in situ conservation Conservation of plant species (domesticated varieties or varieties created by plant breeding) in the environment, in which they developed their particular characteristics on farm conservation maintenance, cultivation and development of PGR (mostly land races and traditional varieties) on agricultural farms.
Why in situ conservation?
In situ conservation is essential because many important characteristics of plant genetic resources can only be maintained in the specific environments where they originally evolved. These environments exert selective pressures that shape local adaptation, resulting in traits valuable for long-term agricultural sustainability.
Moreover, agroecosystems are dynamic systems where new genetic diversity is continuously generated through natural and human-mediated (e.g., cultivation in stressful environments likely induces new mutations) processes. Maintaining in situ populations allows this evolutionary process to continue and contribute to the future breeding potential of a crop.
Preserving living populations in their native habitats provides a form of security backup for gene bank collections, safeguarding against potential losses in ex situ storage. Agroecosystems located in centers of biological diversity serve as natural laboratories for research, offering opportunities to study evolutionary processes, co-adaptation, and resilience under real-world conditions. This because, for example, the diversity of pathogens tends to be higher in the center of domestication of a crop than elsewhere, therefore providing a stronger selection regime.
Finally, in situ conservation is a legal and ethical obligation under international frameworks such as the Convention on Biological Diversity, which emphasizes the need to conserve genetic diversity within natural and agricultural systems.
The goal of in situ conservation with respect to plant breeding can be formulated as follows Simmonds (1962):
…what the breeder needs in the interest of long-term adaptability is a continually replenished store of locally adapted variability. – Simmonds, 1962
Methods of in situ conservation
- Genetic reserves
- Dynamic Genepools/Management (‘Evolutionsramsche’)
- On farm conservation
- Home gardens
Genetic reserves
Genetic reserves are designated areas in which wild plant species grow within their natural ecosystems under protected conditions. These areas serve as long-term repositories of genetic diversity, allowing plant populations to persist across generations while continuing to evolve in response to environmental pressures, pests, and diseases. Unlike ex situ methods that freeze genetic variation at the time of storage, genetic reserves support dynamic conservation by maintaining ongoing evolutionary processes. This makes them particularly valuable for preserving adaptive traits that may be critical for future crop improvement.
These reserves are especially suitable for the in situ conservation of crop wild relatives belonging to the primary and secondary gene pools of cultivated species. Maintaining these relatives in their native habitats ensures the preservation of genetic traits that might otherwise be lost, including resistance to local pests and diseases, drought tolerance, and other ecologically relevant features. Because the value of genetic reserves lies in their ability to conserve evolving populations, it is essential that plant genetic resources (PGR) within these areas are regularly monitored for their genetic diversity. Continuous monitoring helps detect changes over time and informs management strategies to maintain or enhance genetic variation (Maxted, 2003).
Example of a reservoir: Apple tree forests in Kazakhstan
A prominent example of genetic reserves is the Zhongar-Alatau State National Nature Park in Kazakhstan, established in 2010 and covering an area of 356,022 hectares. This park includes wild apple tree forests, which are considered the center of origin for the domesticated apple and serve as a vital reservoir for apple genetic diversity.
Dynamic gene pools
Dynamic gene pools
Dynamic gene pools consist of bulk populations, which are artificially created populations derived from crosses among a wide diversity of existing plant varieties. These populations are grown across multiple sites without intentional selection6, allowing natural selection to occur under local environmental conditions, thus enabling the populations to adapt locally over several generations. This approach of creating evolutionary bulk populations (in German: Evolutionsramsche) facilitates continuous adaptation and evolution of genetic resources. Dynamic gene pools can also play an important role in pre-breeding and germplasm enhancement, as they conserve and provide locally adapted variability valuable for plant breeding programs. Additionally, such an approach broadens the genetic base of breeding populations, although not conservation approaches in the strict sense, aim to increase usable genetic variation within breeding populations.
6 Intentional selection is what plant breeders are doing in modern plant breeding programs based on the estimation of variance components, heritability and breeding value estimation
The establishment of dynamic gene pools typically follows a structured approach: first, a starting population is created through extensive mutual crosses among many diverse lines or populations. This initial population is then phenotypically and genotypically characterized, forming a reference point for future comparisons. Representative subsets of this cross are subsequently grown at multiple locations for more than ten generations to encourage local adaptation. Throughout these generations, populations are subjected only to mild, natural selection pressures, thereby allowing the evolutionary process to operate with minimal human intervention. At each generation, representative seed samples from all locations are centrally collected and stored, while phenotypic and genotypic changes are regularly monitored and documented at predefined intervals.
From a population genetics perspective, bulk populations differ significantly depending on whether the species are predominantly outcrossing or self-fertilizing. Outcrossing species, such as rye, maize, and rapeseed, are relatively easy to generate by simple random mating but exhibit slower evolutionary responses since natural selection acts primarily on individual genes. Introgressions, or gene flow between populations, can have significant impacts in these species, necessitating the isolation of established heterotic groups such as, for example, the Petkus and Carsten pools in rye breeding.
In contrast, the creation of bulk populations in self-fertilizing crops, such as wheat, barley, and lentils, is more labor-intensive due to the necessity of manual crosses. Nevertheless, these populations evolve faster because natural selection acts directly on whole genotypes rather than individual genes, leading to recognizable genetic shifts within just a few generations. Additionally, introgression events are less frequent due to the predominantly self-pollinating nature of these crops.
You should understand and be able to explain the two key points made in the above statements:
- Why are outcrossing species usually easier to cross than inbreeding species?
- What is the underlying genetic explanation that selection in outbreeding crops tends to act on individual genes whereas in inbreeding crops tha whole genome tends to be selected?
- Why is evolution faster if the whole genotype is sequenced. Can you think of a disadvantage?
Historical examples of composite crosses highlight successful applications of dynamic gene pools. At the University of Davis, California, Harlan and Martini Harlan and Martini (1929),harlan_effect_1938 crossed 28 barley lines (15 from the USA and 13 from Europe), producing 378 individual crosses (Figure 16). These composite crosses have since been grown continuously at multiple locations without deliberate selection. A recent analysis of these crosses using whole genome sequencing and genetic mapping identified changes in allele frequencies in response to selection, and also uncovered genes where selection had a strong effect on allele frequencies Landis et al. (2024). Such genes were mainly well known genes with a role in controlling flowering time.
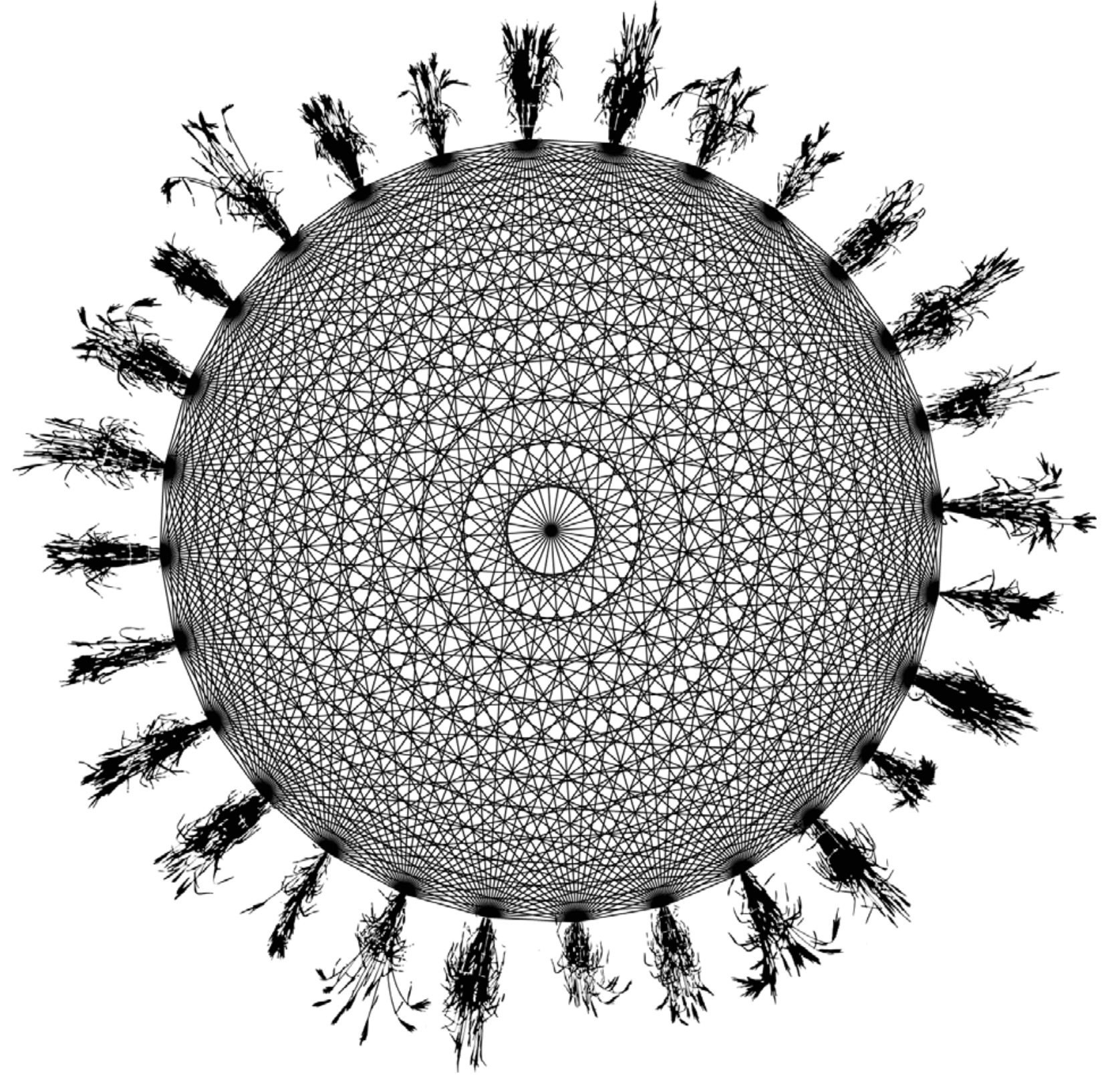
In France, beginning in 1984, Goldringer et al. (2001),goldringer_rapid_2006 generated two populations consisting of two sets of 16 wheat lines (PA and PB) and another of 62 lines (PS), subsequently cultivating these bulk populations across 7 to 12 regions in large plots without active selection.
Similarly, composite crosses initiated in Scandinavia by Veteläinen and Nissilä (2001) in 1991/1992 involved crossing 25 local and 15 exotic barley lines, followed by cultivation at multiple locations throughout northern and southern Scandinavia to promote broad adaptability.
Dynamic management of wheat in France
Paillard et al. (2000) conducted a long-term study on the dynamic management of wheat populations cultivated under contrasting farming conditions across multiple regions in France (Figure 17). The aim was to investigate the evolutionary potential of composite populations when grown under both intensive and extensive agricultural practices. A key trait under observation was resistance to Pseudocercosporella (commonly known as eyespot), a fungal disease affecting wheat. Starting from a baseline resistance frequency of 0.24 in the founding population, the study assessed changes after 10 generations of separate cultivation.
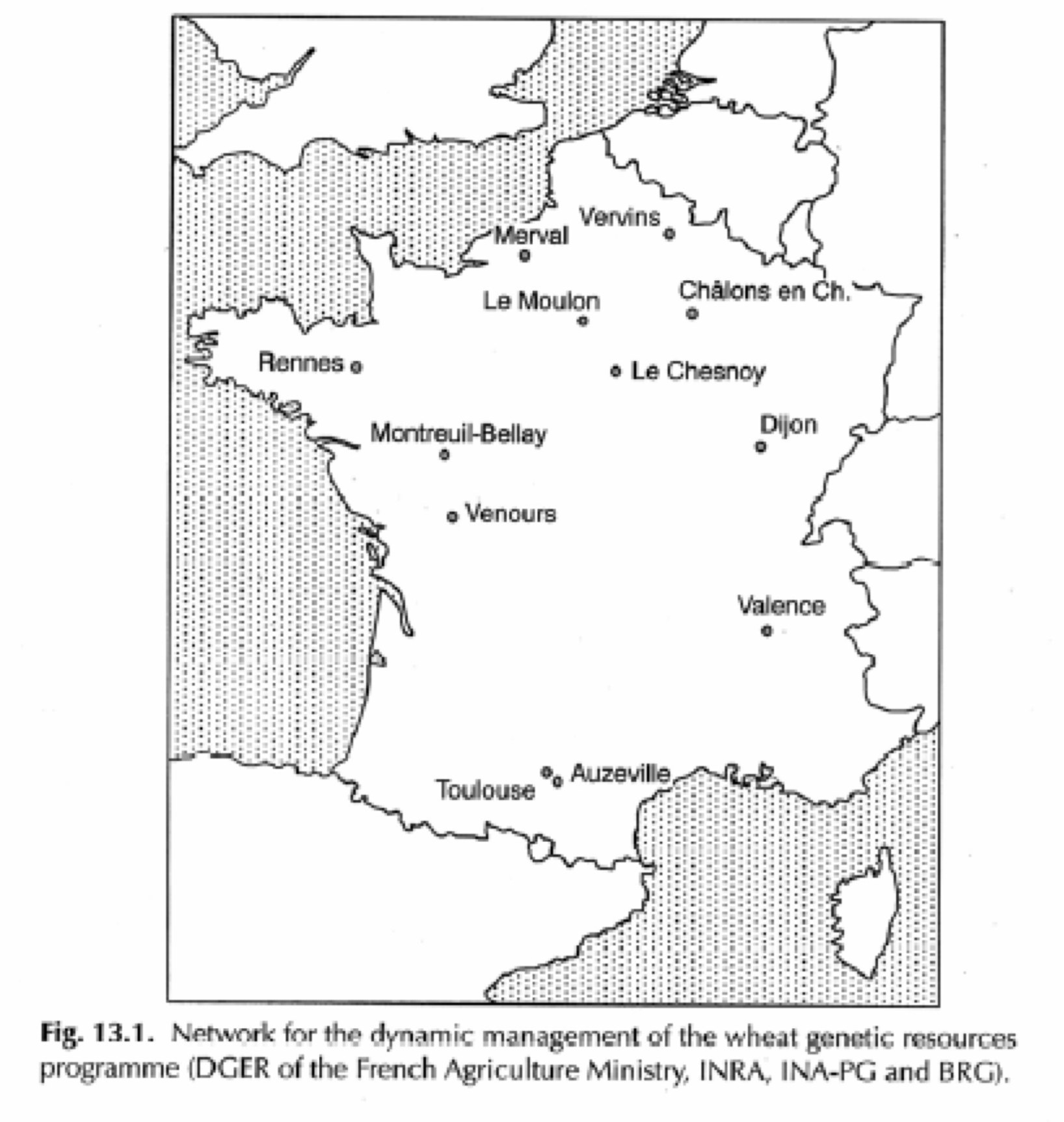
The results revealed substantial variation in resistance frequency depending on the location and management system (?@tbl-goldring2). For instance, under intensive cultivation, resistance frequencies ranged from as low as 0.08 in Montreuil to as high as 0.81 in Vervins. In contrast, extensive management led to a complete loss of resistance in Montreuil (0.00) but relatively high maintenance of resistance in Rennes (0.37) and Toulouse (0.30). Interestingly, the overall average resistance frequency across all locations remained stable at 0.25 after 10 generations, suggesting that localized adaptation processes occurred but did not result in uniform directional selection at the national scale.
Population | Intensive | Extensive |
---|---|---|
Le Moulon | 0.09 | 0.17 |
Montreuil | 0.08 | 0.00 |
Rennes | 0.35 | 0.37 |
Toulouse | 0.11 | 0.30 |
Vervins | 0.81 | 0.23 |
On-farm conservation
On-farm conservation refers to the maintenance or cultivation of crop species by farmers within traditional agricultural systems, as described by Maxted et al. (1997). This approach aims to sustain the dynamic evolutionary and developmental processes that shape the genetic diversity of cultivated crops and their wild relatives under real-world cultivation conditions. It is rooted in the understanding that farmers have historically contributed to the creation and preservation of crop diversity and continue to do so despite ongoing socio-economic and technological changes. The practice emphasizes the crucial role of farmer-led selection, particularly of landraces, in maintaining valuable genetic variation (IPGRI 1998).
Example: Potatoes in the Andes
A prominent example of on-farm conservation can be found in the Andes, where several thousand morphologically distinct potato varieties are cultivated, covering four different ploidy levels. In some Andean villages, farmers grow up to 50 distinct potato types. Despite the availability of modern varieties, traditional landraces remain prominent due to their superior taste, storage capacity, and cultural importance—for example, as traditional gifts exchanged among farmers. These landrace mixtures are not only actively cultivated but also serve as seed sources for future planting, ensuring ongoing conservation (Brush 1995).
Example: Andean root tubers
In another example from the Andes, 12 farming families manage a dynamic mosaic system to conserve a wide array of native tubers. Their cultivation includes 50 landraces of potato, 27 of Oca, 7 of Ullucu (also known as Pappalisa), and 8 of Isano (Terrazas and Valdiva).
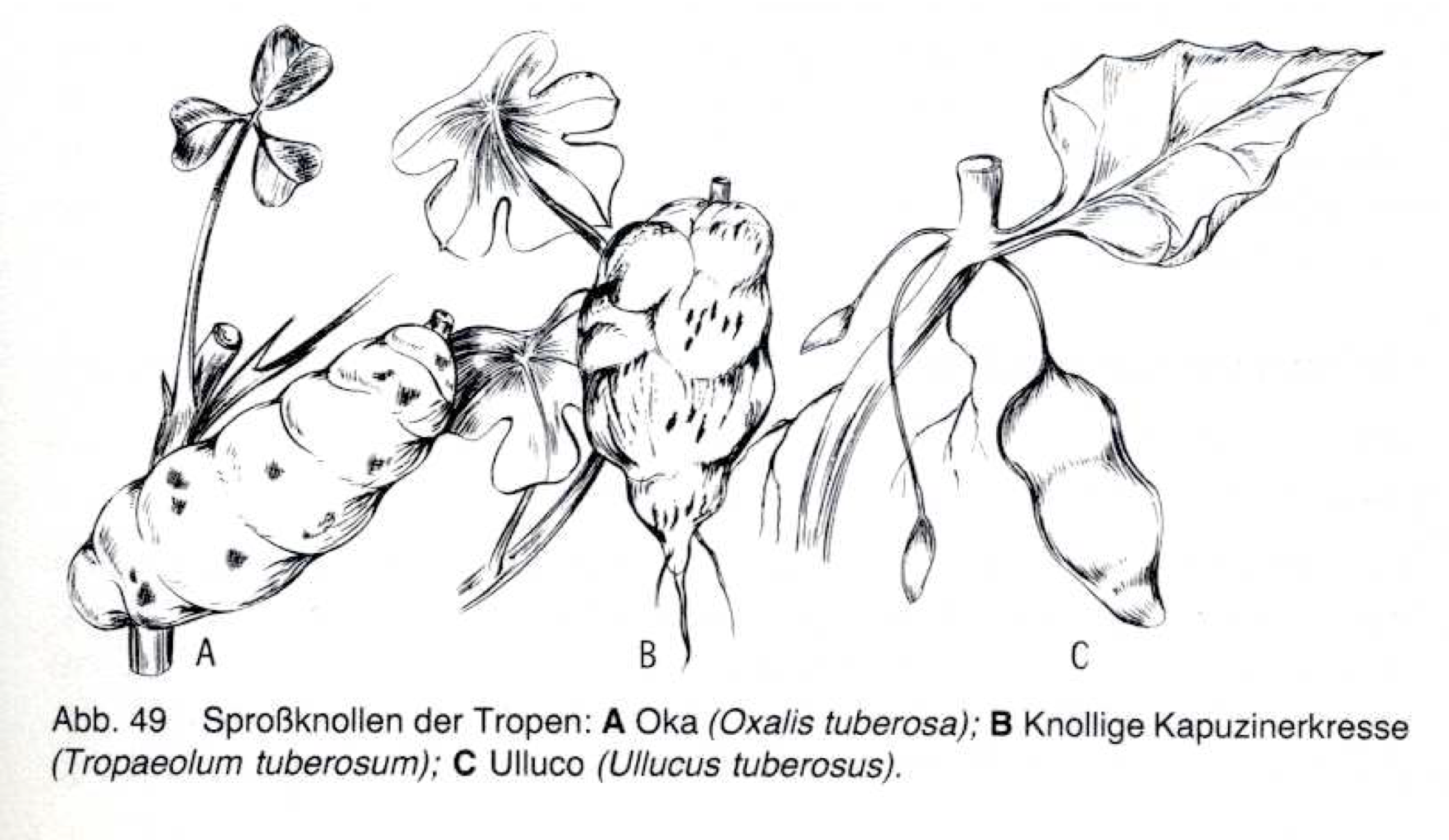
Ullucus tuberosus: Tubers for sale at market, Silvia, Colombia. Photo: Hugh Wilson
Tropaeolum tuberosum – tubers (left) from a Mexican market and (top) plants under cultivation in flower, Lake Titicaca, Bolivia. Photos: Hugh Wilson
Tuber variation of Oxalis tuberosa is also found in markets in Pasto, Colombia, and the flowering plants are commonly seen in Chinchero, Peru.
Mosaic dynamic systems: Parque de la Papa
A well-documented example of community-driven in situ conservation is the Parque de la Papa near Lake Titicaca in Peru.
Mosaic dynamic system: Quinoa in situ conservation
In this system, each family is responsible for maintaining a specific set of quinoa varieties, although the total number of varieties managed in the community has not been formally determined.
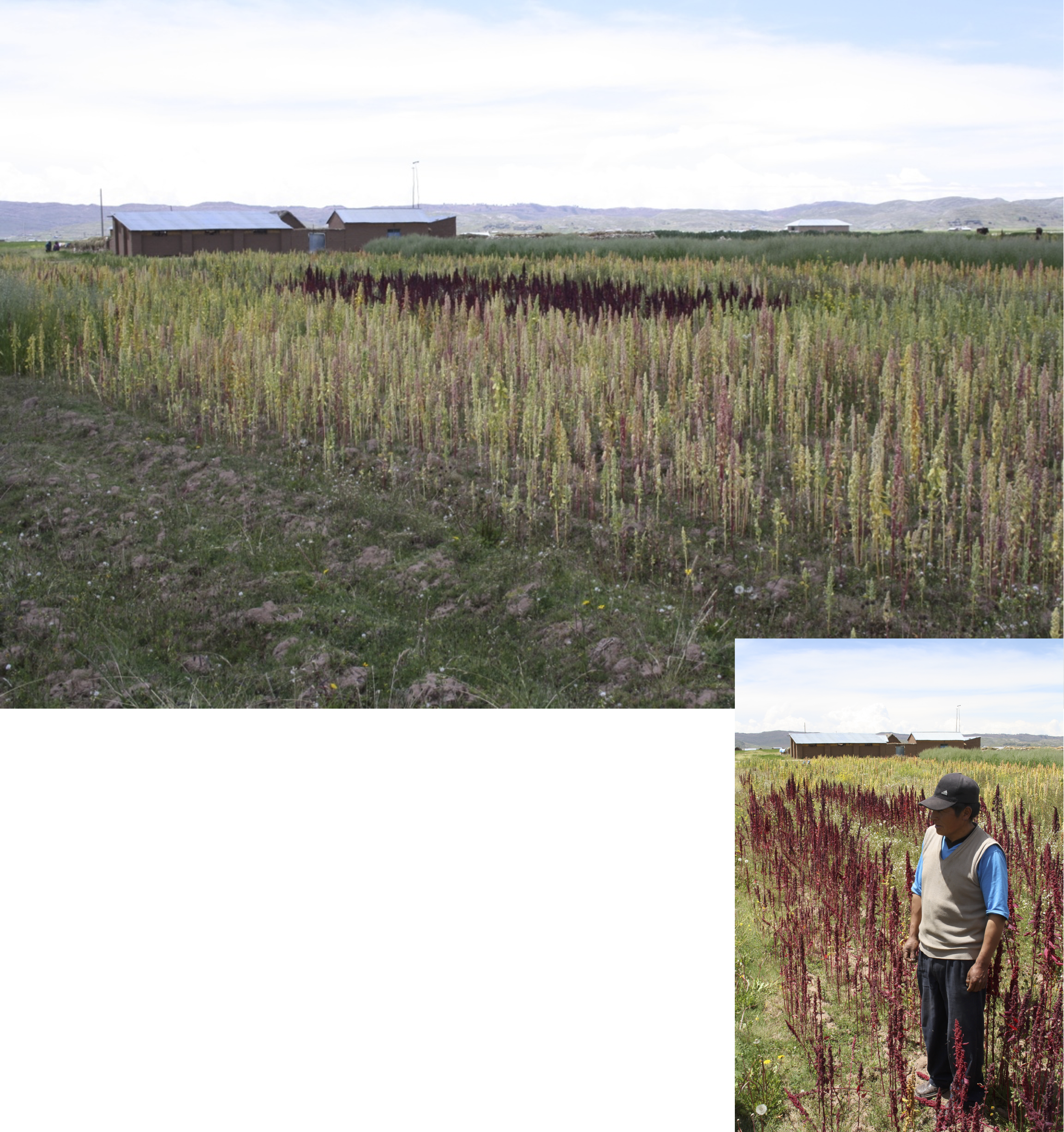
On-farm conservation: Maize in South Mexico
In South Mexico, the diversity of maize varieties has not declined since the 1950s; on the contrary, it has slightly increased. Farmers often integrate modern varieties into their traditional landraces, although hybrid varieties are not widely adopted. A high rate of natural outcrossing contributes significantly to the maintenance and even enhancement of maize diversity in these farming systems (Brush 1995).
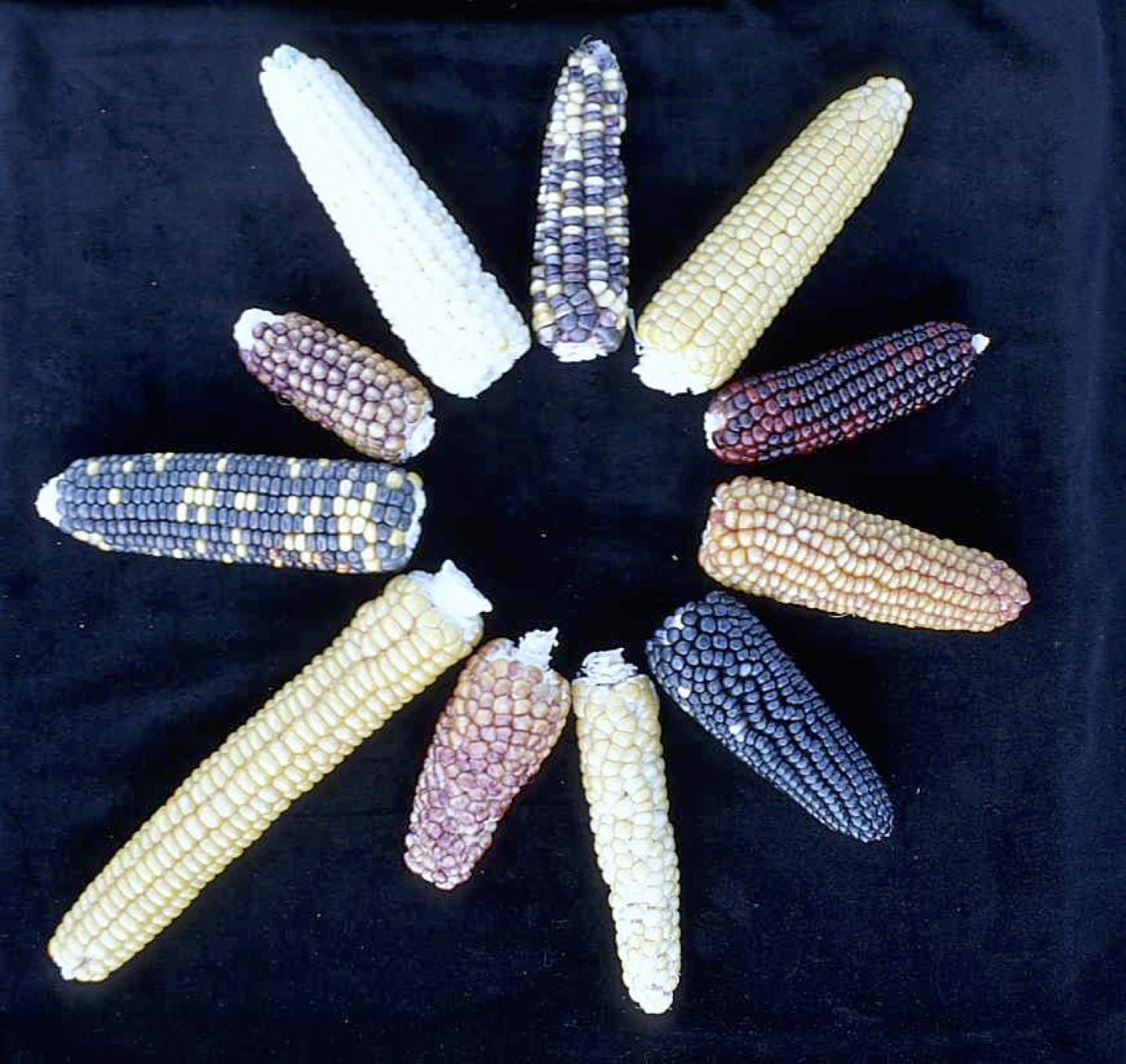
On-farm conservation: Pearl millet in Rajasthan
In Rajasthan, India, pearl millet is primarily conserved through the cultivation of traditional landraces. Farmers emphasize maintaining both genetic diversity and the characteristic traits of specific landraces. These conservation practices differ between regions: farmers in West Rajasthan and East Rajasthan apply distinct strategies to maintain their seed systems and varietal integrity (vom Brocke 2001, PhD Dissertation, University of Hohenheim).
The following table summarizes differences in seed management practices between West and East Rajasthan:
West Rajasthan | East Rajasthan | |
---|---|---|
Goal | Minimize production loss and secure seed availability during droughts | Conservation of specific, morphologically distinct landraces, named after their villages of origin |
Regional level | Frequent seed exchange between neighboring villages, often over long distances | Well-known villages form the basis of local seed markets |
All landraces are seen as equivalent; missing traits do not prevent exchange | Landraces are not exchanged if they lack adaptation or desirable traits | |
Population level | ‘Conservation’ landraces include small amounts of modern varieties (introgression) | Any mixing with modern varieties is avoided to maintain the unique characteristics of traditional landraces |
In situ conservation by state organisations
State institutions also contribute to on-farm conservation. In Peru, the National Institute for Agricultural Innovation (INIA) conducts maintenance breeding of the landrace Blanco de Urubamba. This includes phenotypic selection based on cob morphology to maintain desirable traits across generations.
Home gardens
- Conservation of genetic resources in home gardens is a particular form of on farm conservation
- Home gardens have a high species diversity in small areas
- Species in home gardens are predominately vegetables, tubers, spices and medicinal plants (Tomatos, pepper, maniok, cumin, mint, thyme, parsley)
- Fruit orchards close to homes harbor genetic diversity of fruit trees, wood trees and shrubs
Tropical Home Gardens
Figure 21 shows a tropical home garden in Java, Indonesia.
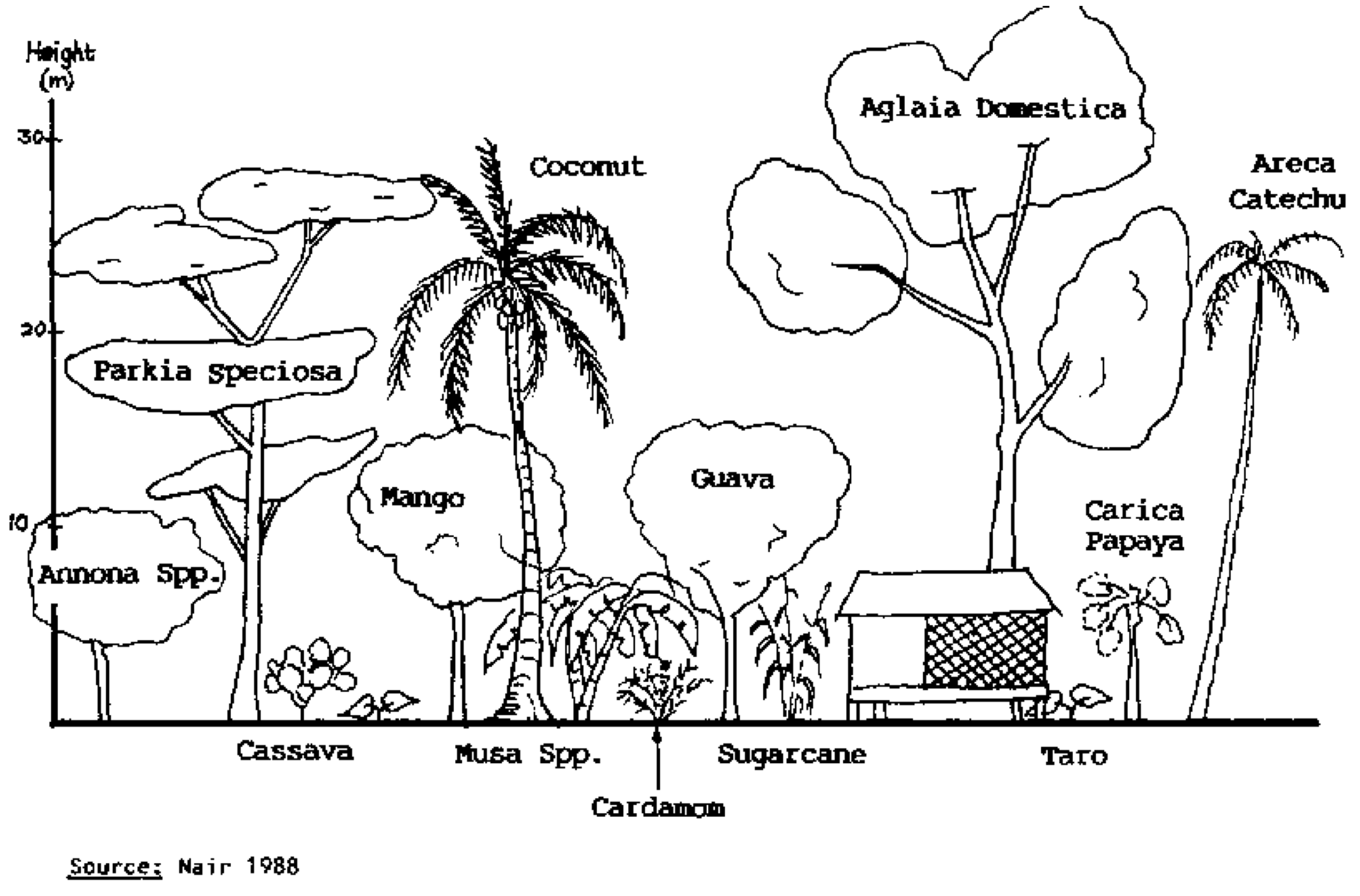
Key concepts
Summary
- Several options are available for managing plant genetic resources.
- The two main approaches for conservation are ex situ and in situ conservation.
- Both ex situ and in situ conservation management can be combined in a single strategy to manage PGR.
- Several options are available for managing plant genetic resources.
- The two main approaches for conservation are ex situ and in situ conservation.
- Both ex situ and in situ conservation management can be combined in a single strategy to manage PGR.
- Genetic erosion is the loss of genetic diversity because of genetic drift. In ex situ collections, there is a significant amount of loss by genetic drift because of the required rejuvenation.
- Different types of in situ management
- Community-driven and state-funded systems
- in situ conservation strategies originated on all continents
- Main goal: keep plants in their native environment
- Effect on long-term trends on genetic variation is unclear
Further reading
Review and discussion questions
- Why are populations with a large effective population size expected to evolve faster than populations with a small effective population size? Why is this relevant in the context of plant genetic resource conservation?
- What are the key arguments against an in situ and against an ex situ conservation strategy, respectively?
- What are the key arguments in favor of in situ and ex situ conservation strategy, repsectively?
- How would it be possible to differentiate between adaptive and nonadaptive traits observed in landraces?
- What are the different types of dynamic gene pools?
- Why is selection in dynamic gene pools slower than in self-fertilizing crops?
- What are the differences between dynamic gene pools and varieties grown in dynamic gene pools?
- What are the challenges of a systematic management of genetic resources in dynamic populations on a national level?
Problems
Discussion questions
- Why are populations with a large effective population size expected to evolve faster than populations with a small effective population size? Why is this relevant in the context of plant genetic resource conservation?
- How would it be possible to differentiate between adaptive and non-adaptive traits observed in landraces?
- Which two main conservation strategies exist and which are their main advantages, disadvantages and challenges?
- Dynamic gene pools
- What are they?
- Why is selection in dynamic gene pools slower than in self-fertilizing crops?
- What are the challenges of a systematic management of genetic resources in dynamic populations on a national level?
Advantages and disadvantages of conservation strategies
There are several strategies for in situ conservation and each have advantages and disadvantages.
Summarize the advantages and disadvantages in the following form:
Ex situ gene banks | In situ on farm | Evolutionary bulks | Genetic reserves | Home gardens | |
---|---|---|---|---|---|
Ability to conserve diversity | |||||
Economic cost | |||||
Description of diversity | |||||
Utilization of diversity | |||||
Monitoring of protection | |||||
Legal protection of diversity | |||||
“Future proofing” |
Interaction between ex situ and in situ conservation
Assume you are given the task to develop a strategy that overcomes the division and disadvantages of ex situ and in situ conservation and to develop a hybrid strategy.
Develop a short plan that would overcome the disadvantages with a reasonable economic cost.