Introduction
Motivation
The motivation for teaching this module as part of the plant breeding major depends on a few observations and developments.
First, world nutrition depends on few crops. Since they are so important, the major crops need to be in good shape: they need to be healthy and high-yielding in a rapidly changing environment. One may also ask whether it is better to cultivate a high diversity of crops instead of only a few.
Second, the human population is still increasing and expected to reach about 10 billion people by the year 2050. For this reason, an increase in yield is required (Ray et al., 2013), and current growth rates in yield are not sufficient to meet this goal (Figure 1). A question in the context of this module is whether plant genetic resources provide a possibility to achieve this goal.
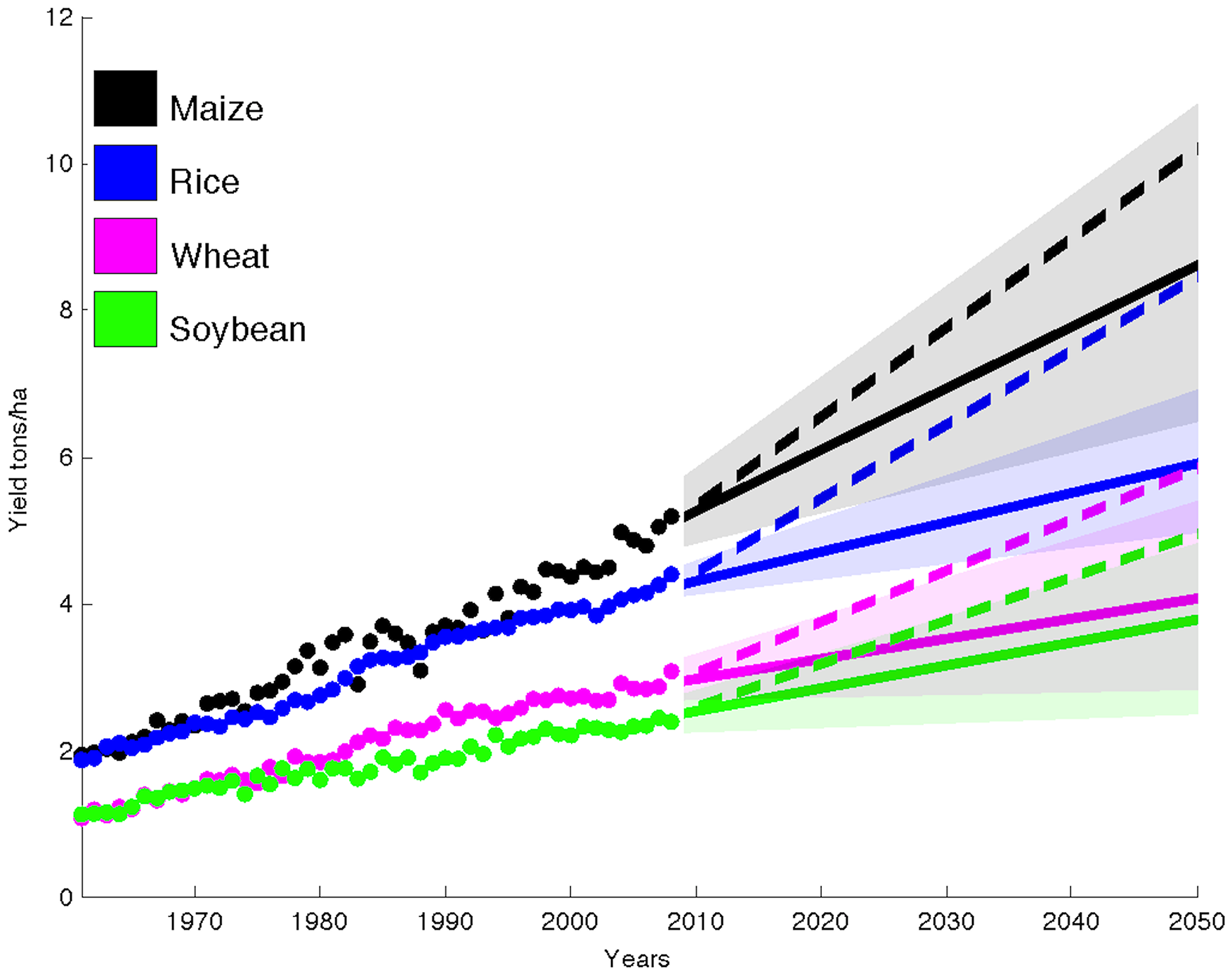
1 See also the global wheat rust monitoring system at FAO
Third, new global threats evolve because of the genetic uniformity of modern crops. In particular, new diseases or new varieties of existing plant diseases may spread rapidly throughout the world and threaten the cultivation of certain crops. A current example is the Ug99 strain the wheat stem rust pathogen Puccinia graminis f. sp. tritici. The map in Figure 2 shows the rapid spread of this strain since its origin at the end of the last century1.
Plant breeders need to react rapidly to this pathogen because no chemical plant protection is available for this pathogen. Genetic resources (i.e., old landraces of wheat or closely related species) have been successfully used to identify new disease resistance genes against the Ug99 strain of wheat rust, e.g., Endresen et al. (2012).
Example: Traditional and modern agriculture in Peru
The contrast between traditional and modern agriculture as well as the role of a highly diverse environment on agriculture and crop plant diversity can be studied in Peru.
Peru is subdivided in three main geographic regions:
- Coastal region (Costa)
- Highland regions and inter-Andean valleys (Altiplano, Valles interandinas)
- Lowlands and Amazonian rainforest region (Selva).
The three regions differ in the type of agricultural systems, crop species cultivated, and varieties of different crops cultivated. The latter differentiation can be demonstrated with maize, which is a very important crop in Peru.
In the coastal region (and increasingly in the rainforest region) modern agricultural production systems are used. Maize is grown as hybrid maize using commercially produced varieties from major international breeding companies. The dominant type is yellow hard maize (Amarillo duro) and it is mainly used for chicken feed.
The original coastal landraces used for human consumption are very rarely grown nowadays.
In the highlands, small peasants use traditional low input agriculture of open pollinated maize varieties, which are highly heterogeneous. In Peru, up to 55 traditional varieties (landraces) of maize are recognized. They were described mostly on the characteristics of their cobs by Grobman et al. (1961) and they are mainly used for human consumption and to a lesser proportion (depending on the landrace) for animal feed (Figure 4).
The differences between the two types of varieties can be seen in Figure 5. The left variety is a modern hybrid variety of the type Amarillo duro. The right variety is the landrace Cusco Gigante (‘The giant from Cusco’), which is cultivated at about 2,500 - 3,000 m altitude near Cuzco in South Peru and is mainly used for human consumption. For this it is cooked in hot water until soft and then either eaten directly from the cob or as individual grains. The name of this dish is choclo.
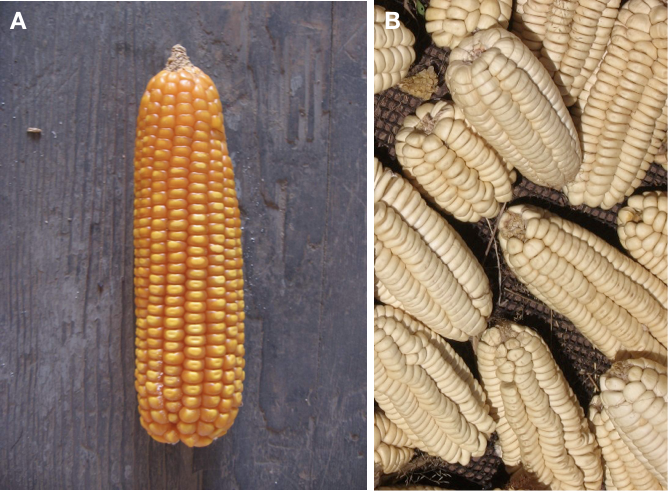
One key characteristic of landrace varieties is the high level of phenotypic and genetic heterogeneity. Figure 6 shows a picture of a gene bank accession of the Peruvian maize genebank at the Agricultural National University, La Molina (near Lima). The picture was taken after collecting the cobs of a landrace in the Ancash province directly from a farmer’s field and it shows the high level of genetic heterogeneity.
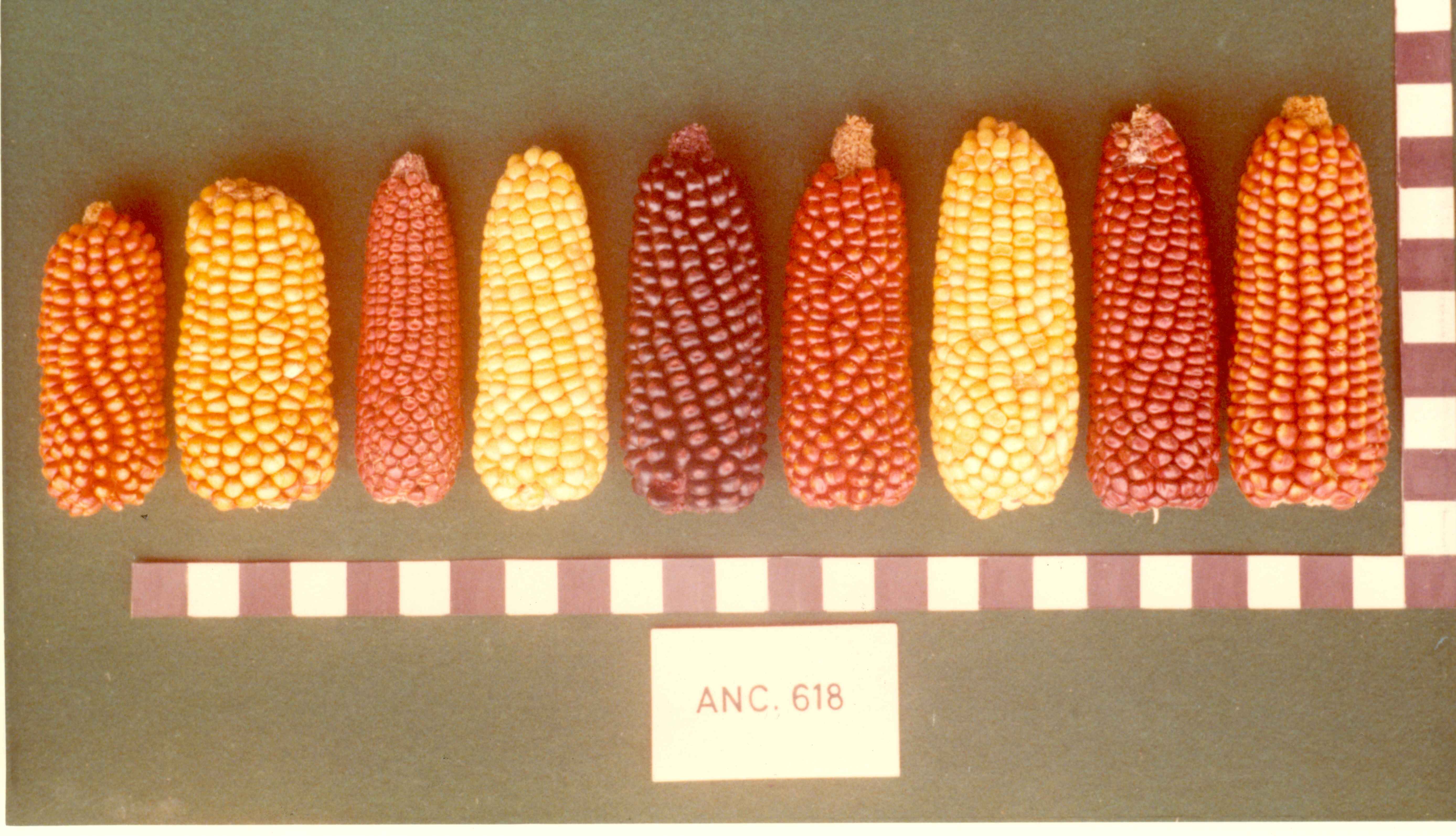
Trade-off between modern vs. traditional agriculture
There is a trade-off between modern versus traditional agriculture that requires to make decisions to ensure food security in future generations. The main cause of the trade-off is the limited area available for cultivation. This requires to increase the yield per area unit to feed an ever growing human population in order to avoid the Malthusian trap, a principle that was formulated by the British economist Thomas Malthus (Wikipedia) (Malthus, 1798). Malthus wrote that because human population growth is exponential, but the growth in agricultural productivity only linear, the increase in agricultural productivity will be insufficient to meet the demand caused by the exponential population growth.
A second source of the trade-off is the ongoing global climate change. Rapidly changing weather patterns require a rapid adaptation of crop plants to future, potentially unknown environmental conditions that can be very different from today. Figure 7 shows that under a 2°C scenario (i.e., a rise of the global average temperature by 2°C until the year 2100, compared to the preindustrial era) the temperature in degrees Celsius of the annual hottest day (i.e., extent of extreme heat), the annual mean total column soil moisture change (i.e., the risk of dry soils) and the annual wettest day precipitation change (i.e., extreme rain events) will all change substantially and to more disadvantageous values in Europe. These models suggest that future crops need to be more heat tolerant, use less available water in the soil and be tolerant to flooding.
From these trade-offs, several questions arise for plant breeding research and the utilization of plant genetic resources:
- Is it better to breed for broad climatic tolerance or narrow local adaptation?
- Should the hundreds and thousands of landraces also be improved by breeding, or should there only be maintenance breeding or no breeding program at all?
- Is the introgression of exotic, adapted material into modern varieties a possible approach for producing ‘future-proof’ varieties?
How important are traditional cultivars?
The Blanco de Urubamba traditional cultivar (“The white from Urubamba”) is similar to the Cusco Gigante variety, but it is cultivated at a lower altitude (>2,000 m altitude). This variety is highly susceptible to the Fusarium pathogen (Figure 8 A), which produces highly toxic compounds in the cob, and it is also susceptible to other diseases. Therefore it should be improved by plant breeding to be useful as human food and animal feed in the future.
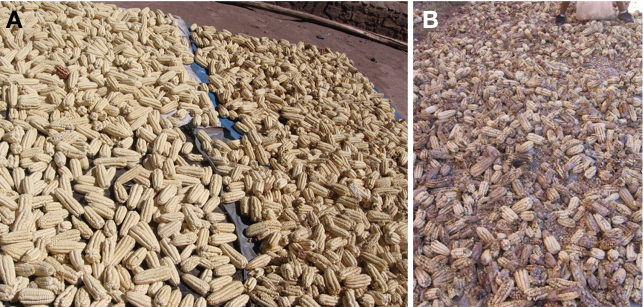
In order not to lose from the harvest, the cobs are visually selected: Beautiful cobs are used for sales and as seeds for the next year, the remaining cobs are select for animal feed (Figure 9 (a)), and then parts of infected cobs or even individual kernels are selected by the local poor (Figure 9 (b)). Most likely, cobs and kernels, which are partially infected are not safe to eat. This selection happens in many locations and with many crops for thousands of years.
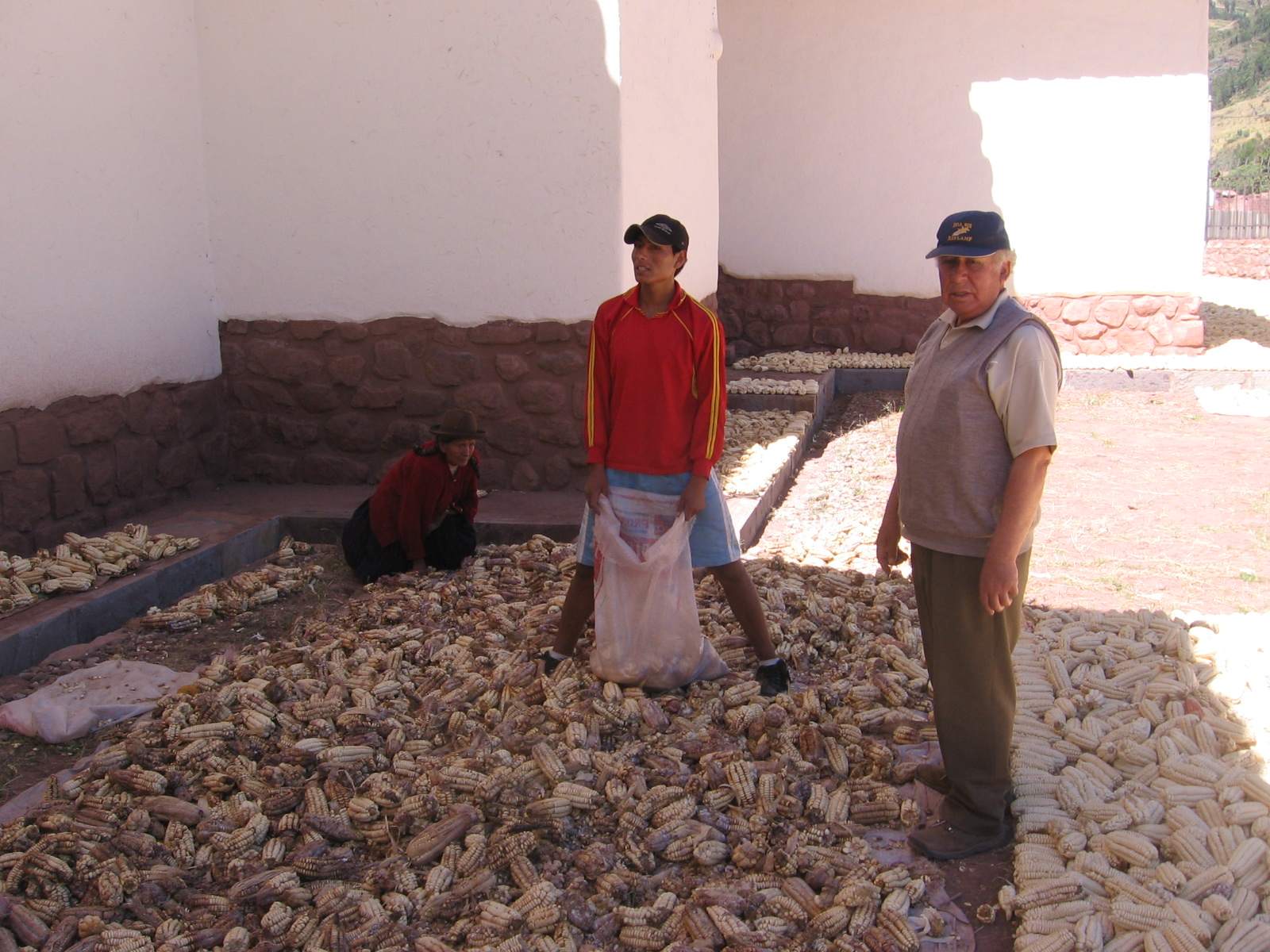
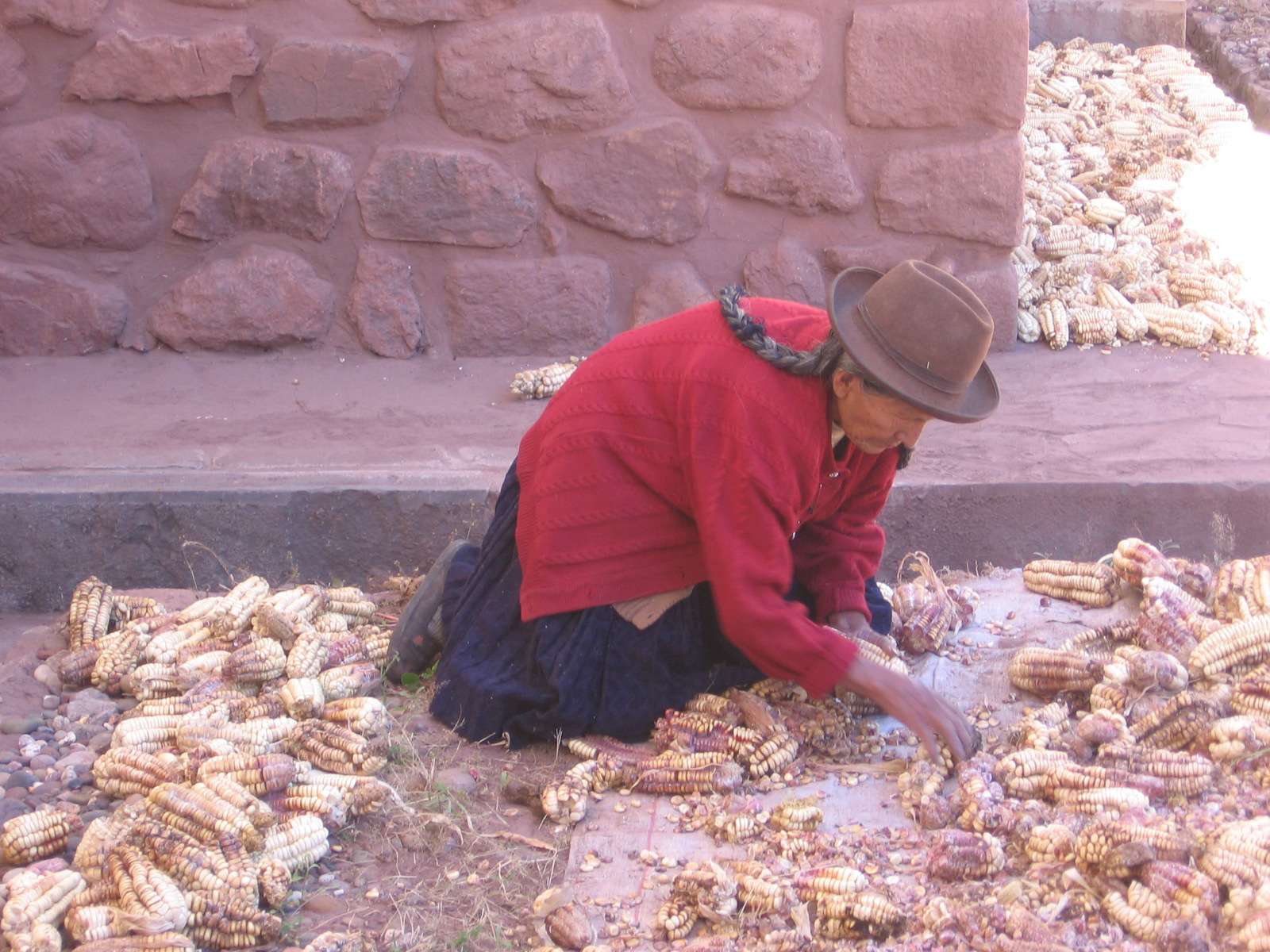
- Selection of cobs and kernels that do not seem to be infected by Fusarium and therefore deemed suitable as animal feed. The picture was taken at the church yard of Taray/Peru in the Sacred Valley of the Incas, where the maize cobs are selected and dried in the tropical sun.
- The remaining cobs are deemed suitable for consumption by humans. Selection of cobs and kernels that do not seem to be infected by Fusarium and therefore deemed suitable for human consumption by the elderly poor. The pictures were taken at the churchyard of Taray/Peru in the Sacred Valley of the Incas, where the maize cobs are sorted and dried in the tropical sun. Photos by Karl Schmid
One solution to overcome the problem of lacking resistance is to develop an improved variety directly out of the landrace by a mass selection approach, i.e., by selecting the most beautiful cobs for seed production (Figure 8), which however is not a very efficient approach for plant improvement.
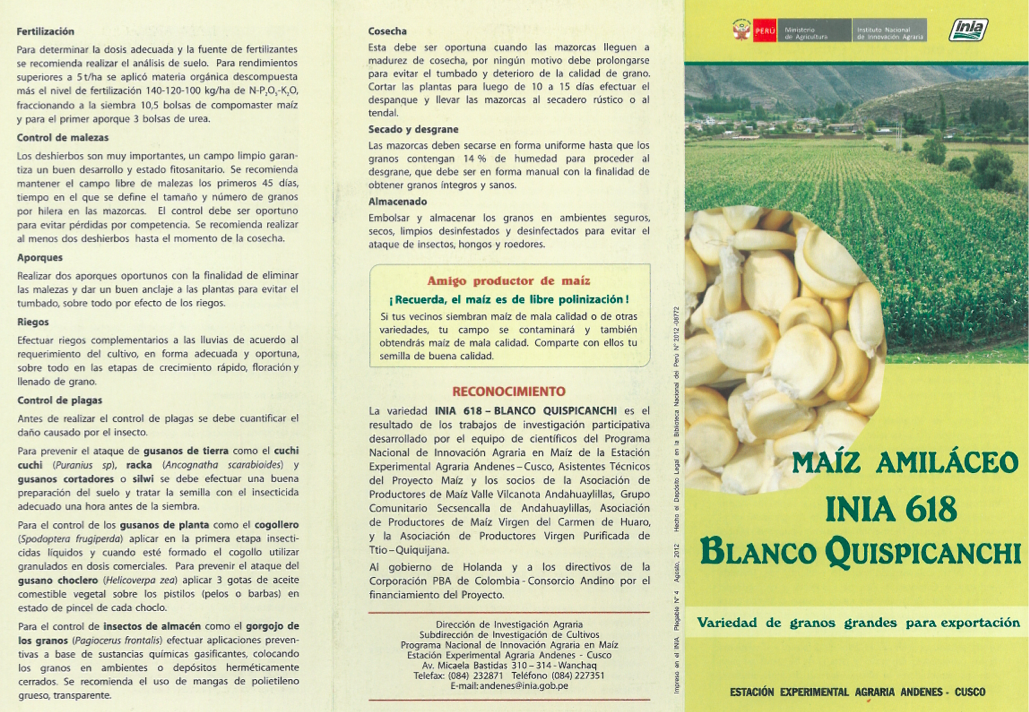
Producing an improved landrace
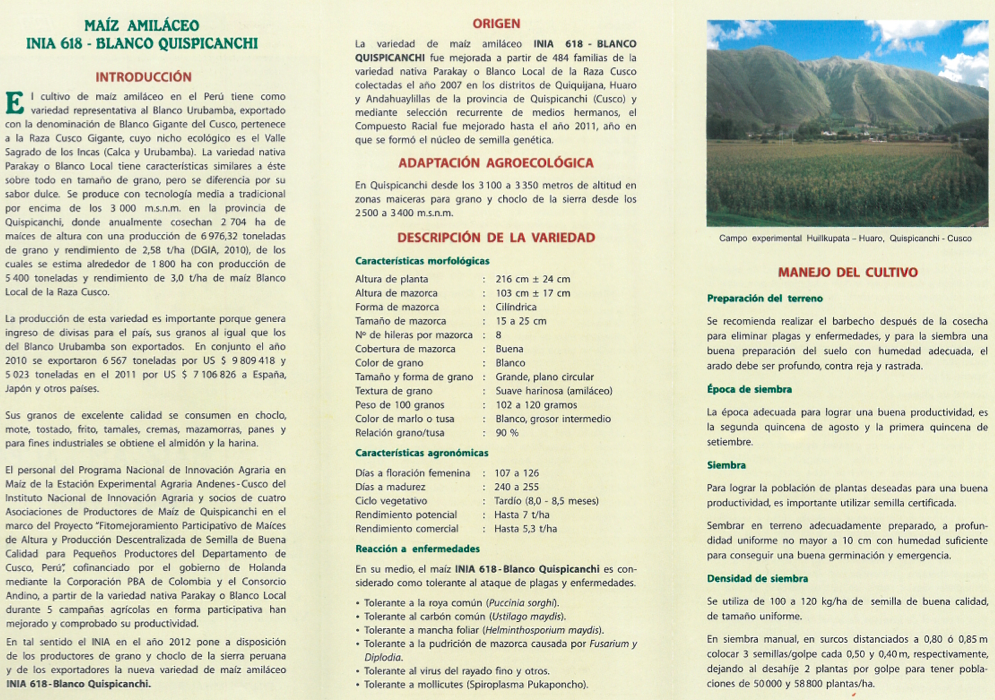
The original Blanco de Urubamba landrace and the improved INIA618 variety are cultivated over large areas in the sacred valley of the Incas near Cusco on 2,900 m altitude (Figure 12). This is essentially a monoculture with a single or very few maize landraces that appear to be quite homogenous.
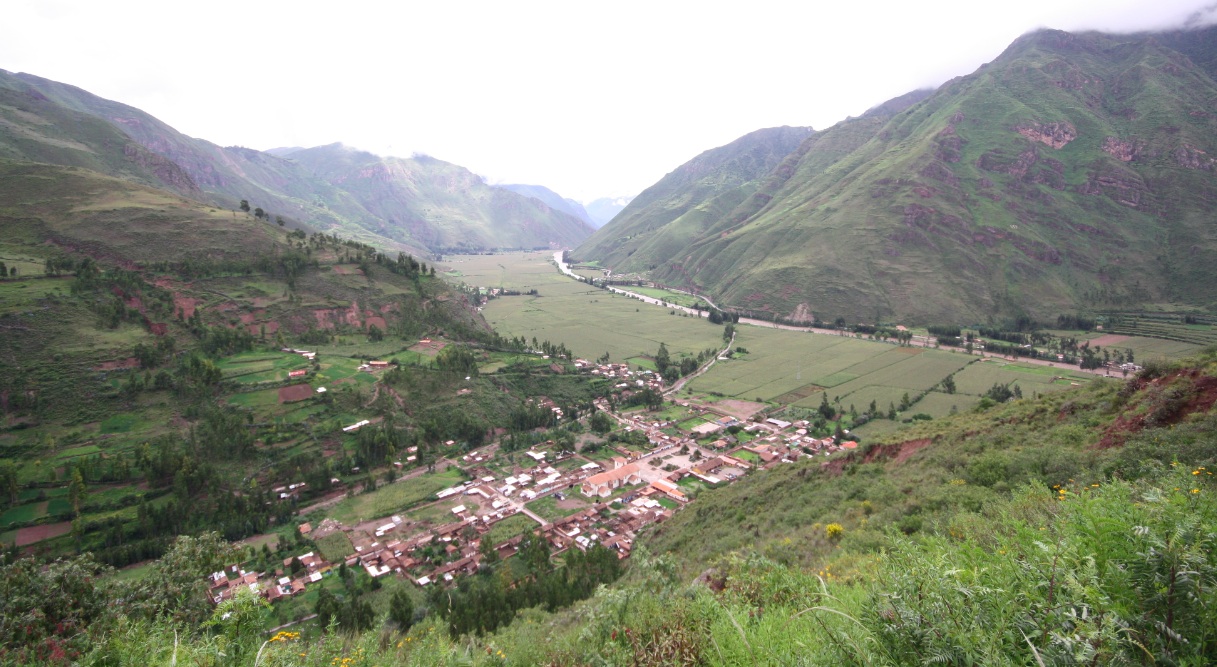
The improvement of a maize variety by a mass selection method such as half-sib selection or full-sib selection results in more homogeneous populations. However, it also suggests that genetic diversity has been lost in the material, and for this reason, may either contribute to inbreeding depression or a reduced adaptability to a rapidly changing climate. The extent of genetic variation in this population is not known as there is no analysis of genetic variation using molecular markers.
Another useful approach is to utilize the specific genetic diversity present in the Peruvian or other native landraces and utilize it for improving modern hybrid varieties cultivated over large areas by using introgression into modern elite varieties. In this case the traditional cultivars are genetic resources to be exploited for useful traits for the development of modern, i.e., hybrid varieties. The preservation of the landrace per se is not a primary goal.
Key goals of the module
Based on the above considerations, the module will explorekey topics essential for understanding and leveraging biological diversity in agriculture, specifically for the development and improvement of crops. Initially, it will delve into the understanding of the different types of biological diversity, aiming to identify which forms are most relevant for the development of new crops and the enhancement of existing ones. This understanding is critical as it lays the foundation for subsequent topics. The module will then cover the methods used to analyze genetic variation, focusing on describing patterns and levels of genetic variation and how these can be utilized to identify valuable genetic traits for breeding purposes. This analytical approach is fundamental in recognizing and harnessing useful genetic diversity.
Furthermore, the module will explore the practical applications of this diversity in breeding programs, discussing how new and exotic genetic diversity can be incorporated into breeding efforts to enhance crop resilience, yield, and nutritional quality. The strategies for collecting and managing genetic diversity will also be addressed, highlighting the importance of preserving genetic resources for future agricultural advancements. Lastly, the module will examine the political and legal aspects surrounding plant genetic resources, emphasizing the significance of these considerations in the context of global agriculture and biodiversity conservation. This comprehensive approach will provide a solid foundation for understanding plant genetic resources, with a more technical definition to be elaborated on in later classes.
Based on these considerations, we can define the topics of the module:
- Understand the different types of biological diversity to understand which one is relevant for the development of new crops and the improvement of existing ones.
- Methods to analyze genetic variation: How can we describe patterns and level of genetic variation and use them for identifying useful genetic variation?
- Utilization of diversity in breeding: How can new and exotic genetic diversity utilized in breeding?
- How to collect and manage genetic diversity.
- Political and legal aspects of plant genetic resources.
A more technical definition of plant genetic resources will follow in the later classes.
Organization of course
The module is roughly organized around three concepts:
- Part 1: Methods for the analysis of genetic and phenotypic variation
- Part 2: Importance of plant genetic resources
- Part 3: Utilization of plant genetic resources
Within those parts there are different topics. For each topic, a motivation is given (i.e., it addresses the question “Why should I learn this?”), a list of key concepts, and review and discussion questions for class. In addition, literature references are given, which include scientific literature, but also more general papers and books that provide a broader context of the topic.
Key concepts
\(\square\) Landrace | \(\square\) Malthusian trap | \(\square\) Major crop |
\(\square\) Open pollinated variety | \(\square\) Mass selection |
Study questions
- What are possible reasons that world nutrition depends on few crops only?
- What is the Malthusian trap and why is it such a powerful metaphor?
- How would you define a major crop?
- What is the definition of a land race? Can you find out, how many landrace of each of the major crops exist?
- If a landrace is improved by breeding (such as the Cusco Gigante landrace) then genetic uniformity may become a problem. Can you explain why uniformity increases, and which consequences it may have for the health of such a landrace? What are possible solutions to this problem?